How additives finish the lubricant
Dan Holdmeyer, Contributing Editor | TLT Lubrication Fundamentals September 2022
Formulators must choose the right combination of additives for the desired performance in an application.
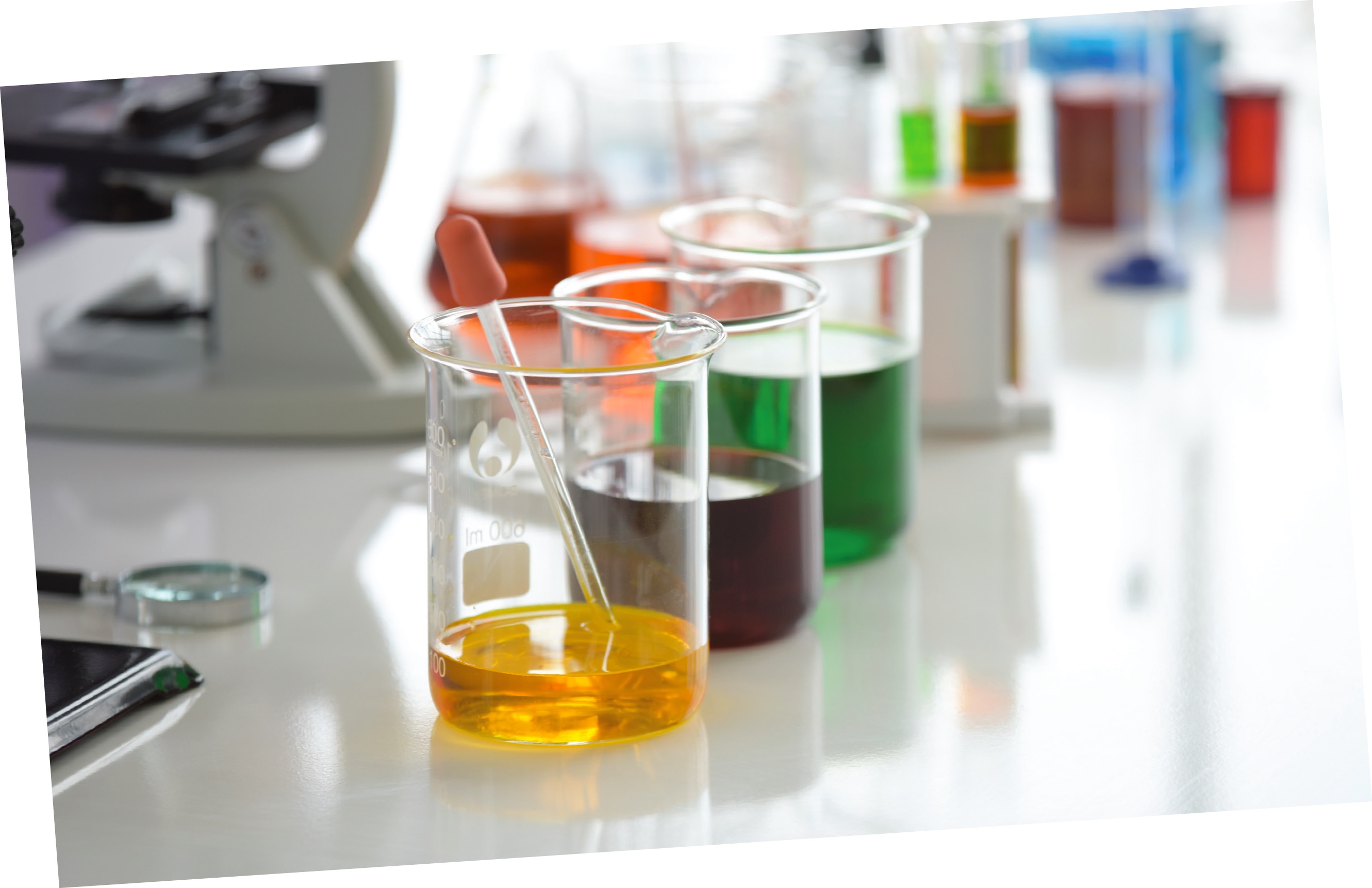
We recently discussed how base stocks are synthesized, or created from crude oil, and how they are categorized. In the early days of crude oil drilling and refining, some base stocks, distilled into appropriate viscosities, were used as lubricants without any additives. Most of today’s lubrication applications require performance characteristics beyond the capabilities of a base oil alone, whether it consists of a single base stock or combination of base stocks.
General performance properties vary with the selection of the API Group base stock. Even within a specific API Group base stock, performance variances exist between refiners. Finished lubricant formulators must choose the base stock combination that provides the base oil performance characteristics to build upon for the desired finished lubricant. The formulators look at the stability of the base stocks used. Base oils are chosen to provide the foundation for stability against oxidation, thermal breakdown and hydrolysis (breakdown by reacting with water). The base oil also provides the minimum viscosity and the lubricant film. Each base stock has various viscosity grades from which a “family” of finished lubricants can be formulated.
Producing today’s finished lubricants for desired performance in an application is a complicated process. Finished lubricants contain specific amounts of selected additives in a particular base oil. Additives either impart new or strengthen existing performance properties of the base oil for the finished lubricant. The base oil is the major component of any finished lubricant. Engine oils have the highest percentage of additives compared to turbine, hydraulic, gear and other non-engine oils.
First, back to my “Ole Plow Boy from Missouri” nature, Figure 1 shows a representation of an additive molecule.
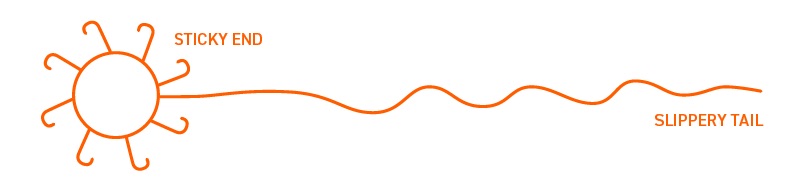
Figure 1. An additive molecule.
The sticky end is the working end of the additive. It is generally polar, surface active and may contain oxygen, nitrogen, sulfur, calcium, magnesium, zinc, phosphorous, etc. The slippery tail is a non-polarized hydrocarbon chain, similar to the base oil, so the additive can be solubilized in the oil.
So, let’s look at the typical additive content and function for various applications without detailing the chemistry of the various additives.
Turbine oils. These are sometimes referred to as R&O oils for the rust and oxidation inhibitors incorporated in these oils. Although their names suggest the rust inhibitors are for protecting the machinery metals, they also help reduce the oxidation rate of the oil. Conversely, the oxidation inhibitors protect the oil but also help protect the metal surfaces. These work synergistically and are common additives in virtually all finished lubricants with the exception of metalworking lubricants, fire resistant hydraulic oils and soluble oils.
Rust results from the attack of water and oxygen on ferrous (iron or steel) metals. Corrosion inhibitors are usually included with rust inhibitors to protect nonferrous metals from the corrosive attack of acidic and chemically reactive elements. Without these inhibitors, metal surface corrosion leads to the loss of metal exposing fresh metal that wears at an accelerated rate. Additionally, metal ions are produced that promote oxidation of the lubricant. To protect the machinery from rust and corrosion, these inhibitors form films on metallic parts to protect them from attack by contaminants in the oil.
Metal deactivators also may be used in these applications. They have a slightly different chemistry but also form films on metal surfaces by combining with the metallic ions formed reducing the catalytic effect on oxidation rate of the oil.
Oil oxidation is a reaction with the hydrocarbons of the oil. The oxidation rate increases with exposure to heat, water contamination, metallic ions and even exposure to light. Excessive oxidation leads to acid formation in the oil, lubricant breakdown, oil thickening, sludge and varnish formation. Oxidation inhibitors slow the oil oxidation rate by interrupting the chain reactions of reactive peroxides formed in the oil.
As with most things in life, too much of a good thing is bad. In the case of antioxidants, too much of certain inhibitors can harm seals. Too much of certain corrosion inhibitors can become corrosive to other metals.
Used oil analysis utilizes several different tests to monitor oxidation and oxidation resistance of lubricants. Acid number and viscosity change tests could indicate when the oil needs to be changed due to oxidation. Used oil analysis of turbine applications also may use tests that actually look at antioxidation additive depletion, varnish tendency or measure oxidation resistance via the Rotary Pressure Vessel Oxidation Test (RPVOT), but lubricant tests are another discussion topic.
As turbine oils, or any circulating systems’ oils, flow through the systems, air is constantly introduced into the oils and can cause excess foam in the systems. Excessive foam can lead to many maladies from overheating, loss of lubricant film, machine wear and fall hazards if the foam comes out onto the floor. Foam inhibitors, or antifoam agents, change the surface tension of the foam bubbles causing them to burst, releasing the trapped air. Foam inhibitors are dispersed in the oil versus being solubilized in the oil and, thus, if the oil is not circulated or agitated for extended periods, the foam inhibitors may settle out of the oil.
The negative consequence of too much foam inhibitor is more immediately noticeable compared to excessive amounts of other additives, as the result is seen within minutes as even more excessive foam.
Pour point depressants were occasionally used in turbine oils made with API Group I base stocks to enable cold temperature operations. As we discussed in last month’s TLT article,1 API Group I base stocks are created using solvent dewaxing to reduce the amount of wax in, and lower the pour point of, the oil. The pour point depressants worked by minimizing the growth of wax crystals forming in the oil as the temperature dropped. API Group II and III base stocks are catalytically dewaxed, eliminating the wax and, thus, pour point depressants are not needed.
Hydraulic oils. Hydraulic systems initially used water as the power transfer fluid. Water was replaced with oil to protect the equipment from corrosion. R&O oils worked well until hydraulic system pressure rose above 1,000 psi. The lubricant film provided by the base oil of R&O oils alone was not enough to protect the vane pumps from excessive wear and premature failure at those pressures.
Antiwear (AW) additives were added to the R&O oil formulation to make AW hydraulic oils. These additives chemically react with the metal surfaces of the system and provide a sacrificial film to minimize metal-to-metal contact under mild boundary lubrication conditions. The film is sheared away and replaced by the oil reducing actual metal wear in the pump and the rest of the system. For years, the most common AW additive was zinc dithiophosphate (ZDTP, ZDP or “zinc”). Today, zinc-free AW hydraulic fluids also are in the market. Some AW hydraulic oils can claim “ashless” technology, i.e., they contain no metal in their additives while still providing the required performance characteristics. Ash content is an indication of the amount of metal elements in the oil and is even more critical in engine oil design, which we’ll discuss later.
With the introduction of AW hydraulic oils, hydraulic system pressures greater than 6,000 psi are possible today. However, caution must be taken to not use too much AW as the additives can become corrosive to soft metal in the systems.
Hydraulic systems are often exposed to water, whether it is from a “wash down,” cylinders submerged digging rock from a quarry pond, etc. Most hydraulic system oils are not changed frequently and, thus, any water that does get past the seals and into the oil needs to be removed. Demulsifiers enable the water to drop out of the oil and be drained from the system reservoir. Demulsifiers are more essential in AW hydraulic oils as the AW additives tend to want to suspend water in the hydraulic oil, and the demulsifiers help counter that and enable water removal.
Cold weather operation is often a necessity for hydraulic systems. The oil viscosity at low temperatures becomes critical to not starve the hydraulic pump of oil. This results in cavitation2 and sluggish operation. Viscosity index improvers (VIIs) are added to make high viscosity index (VI) hydraulic oils. The benefit is similar to multigrade engine oils—they operate at low temperatures during start up but have enough viscosity at operating temperature to protect the equipment from excessive wear.
So far, we’ve covered R&O inhibitors, corrosion inhibitors, metal deactivators, foam inhibitors, pour point depressants, AW additives, demulsifiers and VIIs for turbine and hydraulic applications. Next month we will discuss how these additives are utilized in many other finished lubricants’ typical additive packages in applications that require several other additional additives.
REFERENCES
1. Holdmeyer, D. (2022), “Crude oil to base stocks to base oils to lubricating oils,” TLT, 78 (8), pp. 24-28. Available here.
2. Holdmeyer, D. (2022), “Air entrainment, aeration, cavitation and foaming: How are they related?” TLT, 78 (2), pp. 24-26. Available here.
Dan Holdmeyer is retired from Chevron Lubricants and is based in Washington, Mo. You can reach him at dan.holdmeyer@gmail.com.