Solid coatings: Not just for space vehicles anymore
Dr. Nancy McGuire, Contributing Editor | TLT Cover Story October 2020
Simple and complex solids provide efficient, cost-effective environmentally friendly lubrication and wear protection for an increasing number of applications.
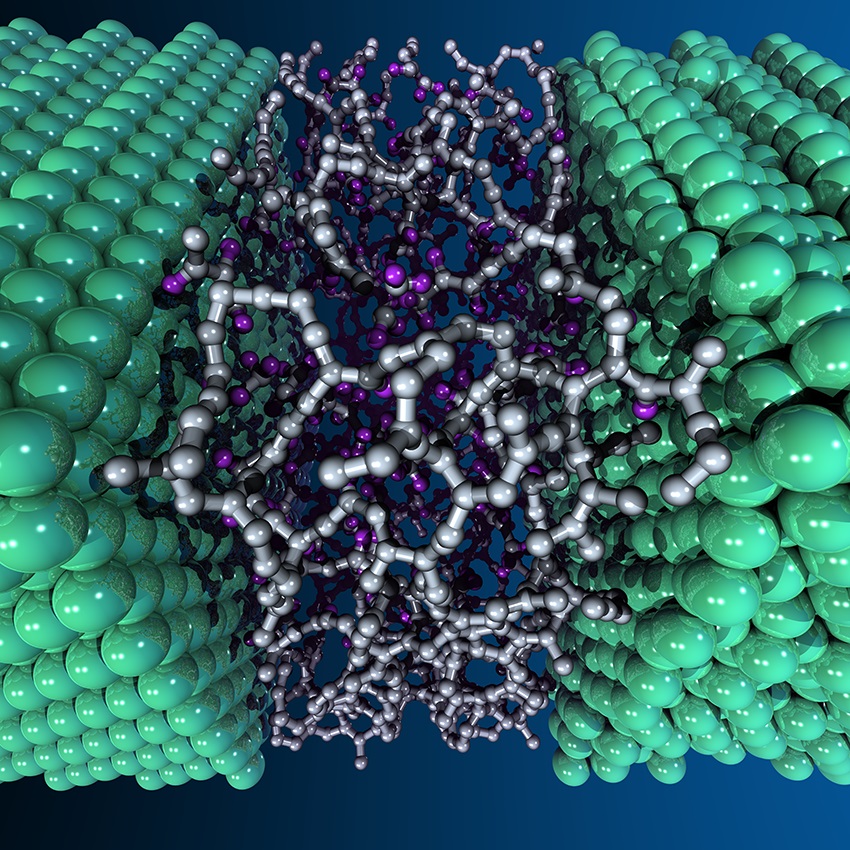
KEY CONCEPTS
•
Solid lubricant and wear protection coatings, once used mainly for aerospace applications, are increasingly used in a wide range of terrestrial products.
•
Some thin solid coatings form self-replenishing lubricant films and last for the lifetime of the devices they protect.
•
Coatings under development use environmentally benign components and processes, and the small amounts used result in less waste.
Solid lubrication and wear reduction coatings were once the purview of NASA and companies in the aerospace industry. Now, they are showing up in an increasing number of applications here on the ground. The component materials for these coatings come from all over the periodic table. “Even with a relatively small number of materials, once you mix three or four things together, the matrix of what you can do is just immense,” says STLE-member Jeff Lince, principal at Space Tribology Consulting.
Varying the coating’s morphology—amorphous or crystalline, macrocomposites, nanocomposites—creates even more options. Some coatings interact with their surrounding environments and each other to form tribofilms or nanoparticles that can almost eliminate the friction between two surfaces.
The applications for these new coatings, some still in the research phase and some already on the market, are just as varied. Solid coatings protect surfaces on everything from razor blades to Ethernet cable connectors to aircraft turbines, and coatings for advanced electrical power generators and medical devices are just over the horizon.
New uses for familiar materials
Graphite and molybdenite (the naturally occurring form of molybdenum disulfide, MoS
2) have been used as lubricants since before the Industrial Revolution. Molybdenite looks like graphite, and it might have been mistaken for graphite in early applications, according to high-temperature solid lubricant pioneer Harold Sliney.
1 These materials continued to be the most frequently used inorganic solid lubricants during the 19th and 20th Centuries.
Over the past several decades, there has been a gradual increase in the performance of MoS
2 lubricants, says STLE-member Harpal Singh, a principal scientist at Sentient Science. MoS
2, which forms a layered crystal structure (
see Figure 1), is used to coat bearings and sliding elements. It works well in a vacuum, but oxidizes in air, which causes its lubrication properties to deteriorate. He notes that interest in MoS
2 coatings for use in space vehicles has increased, but these coatings are expensive, partly because they are applied using physical vapor deposition rather than spread onto a surface as bonded coatings. Research into using dopants like metal oxides, carbides and nitrides in MoS
2 is aimed at improving its performance in humid environments; Singh has investigated doping MoS
2 with titanium to produce a coating that performs better than a conventional base oil.
2
Figure 1. Molybdenum disulfide forms a layered structure (yellow = sulfur, purple = molybdenum). Figure courtesy of Jozef Sivek, CC BY-SA 4.0.
The way a coating is applied to a surface plays a big role in its performance.
3 “Adhesion is always a big thing,” says Lince. “If your coating doesn’t stick to the substrate, all is lost.” Application methods also can extend the range of operating conditions and environments for a given coating. For example, co-sputtering MoS
2 with a metal like gold or an oxide like Sb
2O
3 produces a coating that is more stable than pure MoS
2 for storage or use in air. Lince notes, however, that these coatings still do not perform as well in air as some other available coatings.
Boron compounds, some of which form graphite-like layered structures, are another option. Boron oxide is being investigated for use in environments that are too wet for diamond-like carbon (DLC), or even graphite, to work well, Lince says. In water, boron oxide converts to boric acid, which is a good aqueous lubricant. Commercial borate-based additives (e.g., borate polyols and polyamines) for liquid lubricants are touted for their environmentally friendly properties, and they form resilient lubricious films on load-bearing engine parts. Nanoparticulate boric acid, blended into a suspension with synthetic liquid lubricants, has even better lubricating properties.
Carbon in many forms
Carbon-based coatings come in a wide range of compositions and morphologies that function well in different environments. “You think, ‘how complicated could carbon be?’ but you can form a variety of different properties by varying the hydrogen content in diamond-like carbon, the chemical structure [the ratio of diamond-like to graphite-like bonding], and you can dope it with various things,” Lince says. Coatings made from DLC, an amorphous form of elemental carbon, have been on the market since the 1990s. DLC not only lubricates the interface—it also can protect the underlying surfaces from wear.
Solid carbon coatings, used alone or in conjunction with engine oils, reduce friction in electric vehicle drivetrains as well as in some internal combustion engines, says Lince. In high-end vehicle engines, DLC coatings are used for pistons, piston rings, tappets and other components. The vast majority of DLC coatings used terrestrially have a lower hydrogen content than the highly hydrogenated DLC coatings that provide extremely low friction in the vacuum of space. Low-hydrogen DLC coatings, some of which have been commercialized, work well in air, as long as some moisture is present, he says.
DLC coatings provide scratch and wear resistance for the glass plates on barcode scanners, high-end watch faces and decorative glass. Razor blades with DLC coatings provide a close shave without chafing the skin, and they help blades last longer by protecting them against corrosion. DLC coatings also protect metalforming tools and firearms. DLC’s resistance to wear, and its hardness, low friction coefficient and biocompatibility, makes it a popular option for coating surgical instruments. In the food industry, DLC provides inert, non-toxic mold-release coatings, and DLC gas barrier coatings keep carbon dioxide in and oxygen out in thin-walled PET polymer beverage bottles. DLC coatings on wind turbine gears, sliding components and rolling elements help to prevent wear, micropitting and smearing.
Doping DLC with nitrogen forms a material commonly referred to as carbon nitride, which combines the properties of DLC with those of hard ceramic nitrides. Nitrogen doping lowers the film stress so the coating can withstand higher loads and last longer. These coatings, Lince says, are sometimes used on sliders and disks in mechanical computer hard drives to protect the metal parts from degrading in the presence of perfluoropolyether (PFPE) oil lubricants.
Solid lubricants have several environmental benefits, says Anirudha Sumant, who leads a group at the Nanoscience Center for Nanoscale Materials at Argonne National Laboratory. His group spends much of its time developing energy-efficient systems. Lubricants made from graphene and other 2D materials factor heavily into this research, as do diamond and diamond-like carbon.
In addition to reducing fuel consumption costs by reducing friction, these lubricants reduce disposal costs because of the small amounts used and their long service lifetimes. Elemental carbon coatings eventually degrade into graphitic carbon and do not pose the serious chemical pollution problems that petroleum-based lubricants do, he says. “We’re not going to replace oil everywhere,” he adds, but for bearings and many other applications, solid lubricants could one day replace oils and greases.
Graphene, first isolated in 2004, is well-known for its excellent electronic properties. However, its extreme mechanical strength, its lubricious properties and its effectiveness as an antiwear agent were discovered only recently, Sumant says. Thin graphene coatings, only a few atomic layers thick, are impermeable to liquids and gases, a useful property for protecting against oxidation and corrosion. Graphene’s surface is naturally atomically smooth, and graphene flakes in solution conformally coat microscale and nanoscale objects.
4 Under certain environmental conditions, some graphene-based coatings have achieved superlubricity, where the coefficient of friction is close to zero.
Graphene is surprisingly sturdy and effective at reducing friction between steel surfaces in dry and humid environments, Sumant says. This is a notable contrast to graphite or DLC, which work best only in specific environments. The strength of the van der Waals forces that bond graphene’s single carbon layer to the metal surface enable the base layer to undergo almost 50,000 shear cycles before wearing away. A second, mobile graphene layer on top of the first one provides the lubricating effect. Fortunately, even less expensive grades of graphene work well as lubricants, he adds. Oxygen groups on the surface of less-expensive graphene help it bond well with the substrate, and they provide bonding sites for other components of the coating or lubricating particles.
Graphene can be made more hydrophilic by oxidizing the surface, Lince says. Graphene oxide, combined with a polymer composite, could be useful for reducing friction in water-based environments. Researchers also are looking into the effect of dopants like fluorine and hydrogen on graphene’s tribological properties. Lince cites earlier research showing that fluorinated graphite (graphite fluoride) provides better lubrication than graphite does in dry and vacuum environments, and he says the same might be true of fluorinated graphene.
Graphene’s extreme mechanical strength, its lubricious properties and its effectiveness as an antiwear agent were discovered only recently.
Nanodiamonds, another component of Sumant’s research into superlubricity, are made using a well-known commercial process in which TNT wrapped around graphite is detonated to produce a shock wave. The combination of high temperature and pressure converts the graphite into inexpensive nanoscale diamond particles. “The size of the nanodiamonds produced during this process works perfectly well” for making superlubricants, Sumant says. “Larger diamond particles tend to develop crystal facets and will be more abrasive and therefore do not work.”
Sumant’s group also published the first demonstration of superlubricity in macroscale systems, capable of undergoing standard pin-on-disk tests.
5 They found that, at a sliding interface between a DLC ball and a flat surface covered with graphene flakes and nanodiamonds, the graphene bonded to the nanodiamond surfaces, wrapping around the nanodiamonds like scrolls to form nanoscale “ball bearings” (
see Figure 2). More recently, they observed that under dry conditions and high contact pressures, nanodiamonds react with sulfur released by MoS
2, forming onion-like layered carbon structures (
see Figure 3). They patented this material in August.
7
Figure 2. In a dry environment, a graphene scroll forms around a nanodiamond, reducing the friction between a graphene surface (bottom) and a DLC sliding element (top). The scrolls do not form in a humid environment. Figure courtesy of Argonne National Laboratory.
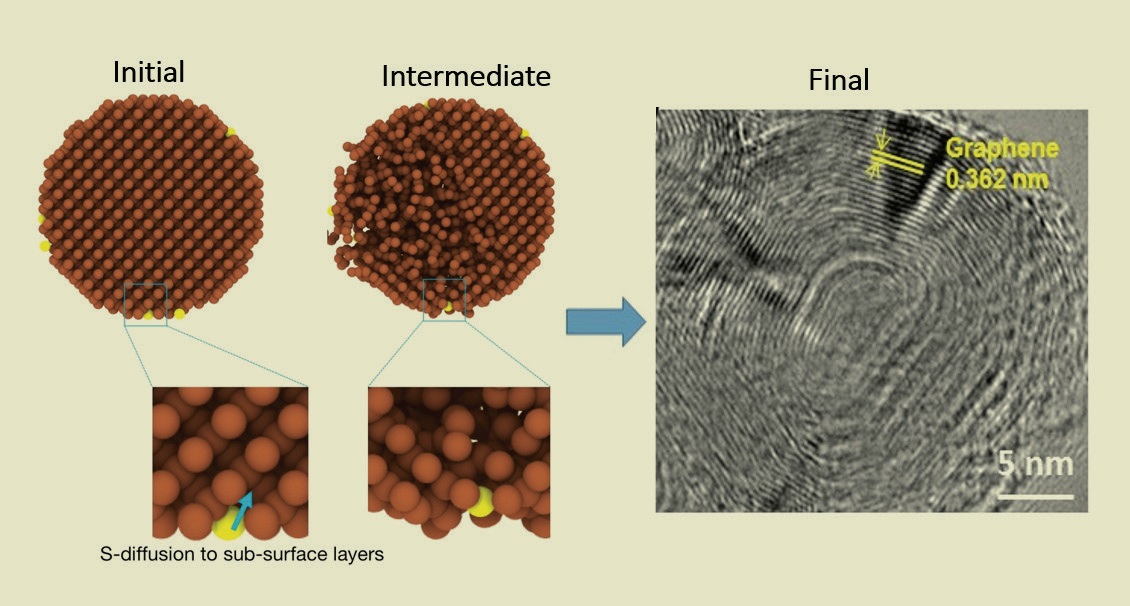
Figure 3. Onion-like layered carbon structures (right), a novel type of superlubricant, form when sulfur from MoS2 diffuses into nanodiamonds under high sliding contact pressure. Figure courtesy of Argonne National Laboratory.
Sumant’s team is currently working with representatives from the oil and gas industry to develop and commercialize one of their superlubricant materials for use in leak-proof rotating seals. These seals, typically made from polished silicon carbide, are used for off shore production operations, where the need to minimize downtime and part failures is especially acute. Diamond friction-reducing coatings had been investigated, but they were cost prohibitive at the large scale required. Sumant’s group and an industry partner are adapting one of the Argonne group’s superlubricating materials to the company’s specifications and operating conditions.
Metals and conductive materials
Metal coatings for electrical contacts, used in everything from cell phone charging ports and Ethernet cable connectors to slip rings for satellites and wind turbines, are a major focus of STLE-member Nicolas (Nic) Argibay’s work as a staff scientist at Sandia National Laboratories. Charging ports for electric vehicles, for example (
see Figure 4), require protection against scratches and exposure to rain and dust over a wide range of temperatures. They also must withstand wear and tear from frequent handling by human beings: “User friendly and user resistant,” as Argibay puts it. Like conventional lubricants and antiwear materials, low-shear-strength metallic coatings must protect the underlying metal surfaces, but they also must be electrically conductive.
Figure 4. Plug-in connectors for electric vehicles require protective coatings that resist wear in all kinds of weather. Figure courtesy of Paul Sladen.
Gold and gold-based alloys are commonly used for electrical connectors. Over the past two decades, the electronics sector used between 265 and 280 metric tonnes of gold each year, with the annual cost of raw materials alone topping $1 billion.
8,9 However, the investment pays off in longer service life and less down time, especially for critical components like slip rings (
see Figure 5) in high-end systems, Argibay says. For example, gold slip rings play an important role in transmitting power and data signals from a wind turbine’s generator, gearbox and main bearing to the motors that control the pitch of the blades. If the slip ring fails, the wind turbine shuts down. Unscheduled maintenance for a 10-story turbine located 100 miles offshore is costly in terms of downtime, access to spare parts, transportation for parts and personnel and payment for qualified technicians who can perform the repairs in hazardous conditions.
Figure 5. Slip rings on a hydroelectric generator.b(A) stationary graphite brushes, (B) rotating steel contact ring, (C) insulated connections to generator field winding, (D) top end of generator shaft. Figure courtesy of Wtshymanski, CC BY-SA 3.0.
“Our team spent a great deal of time, particularly understanding the failure mechanisms of these metallic coatings,” Argibay says. “These gold alloy coatings have been used for many, many decades now; they’ve improved, but it’s been rather largely an incremental improvement if you continue to use these gold-based alloys.” His team has uncovered some of the underlying mechanisms for coating failures, wear, performance margins, how much force you can apply and the conditions the coatings can survive. He notes that finding non-incremental opportunities for improving performance is becoming more complicated, requiring interdisciplinary efforts and more attention to synergistic effects.
Automotive applications
Tribological systems aren’t just about the materials, Argibay says. You also have to take the operating conditions into account. He notes that sensors currently in use for electric cars and other vehicles, wind turbines and other mechanical systems provide a rich stream of information on operating conditions, such as temperatures and vibrations. Installing sensors to collect and process data specific to friction and wear could enable access to a data stream from vast numbers of consumer devices, like electric vehicles. This would, in effect, be crowd-sourcing field testing, using thousands—or millions—of systems under real-world operating conditions. “Every vehicle could be a tribometer,” he says.
Superlubricants could one day prove useful in automobile manufacturing, especially as the industry increasingly shifts to using strong, lightweight alloys. High-temperature metal stamping is used to manufacture car chassis skeletons, Sumant explains. At elevated temperatures, friction between the chassis part and the stamping press causes pinching, which produces thinner, weaker areas in the finished part, he says. Oil lubricants are poorly suited for operations above 500 C, and any type of liquid lubricant must be thoroughly washed off the metal surface before the part can be painted, which greatly increases production costs. Graphene-based lubricants work well in the temperature window required for metal stamping operations, and over the past two years, development efforts have made progress toward making these materials on a production scale, he adds.
Turbines, large and small
Turbines, another application area for solid coatings, are essential to electrical power generators, jet engines for aircraft and air circulation systems. For example, the same solid coatings can protect gears and bearings in offshore and landbased wind turbines, Singh says. Offshore turbines, which are constantly exposed to moisture and salt spray, especially benefit from strong, wear-resistant coatings that also provide some protection against corrosion. Offshore turbines, which tend to be much larger and more remote than their land-based counterparts, are more expensive to maintain and repair. Thus, even expensive coatings can reduce costs by extending the service life of their mechanical parts, he adds.
Friction-induced electrostatic discharge can be a problem for wind turbines, Argibay says. Wind turbines must be built to withstand static charge buildup and even lightning strikes. The solid coatings and lubricants that separate the metal surfaces can either provide electrical insulation or provide a better conductive pathway to ensure that charges don’t build up to the point where they cause damaging arc discharge across bearings, he adds.
Aircraft turbine engines, another major area of application, operate most efficiently when the clearance between the turbine blades and the engine housing is very small to minimize the amount of gases escaping around the edges, Lince says. Using abradable sacrificial coatings, made from solid lubricants mixed with a metallic or ceramic base material, prevents turbine blades from wearing if they come into contact with the housing. Graphite mixed with metals provides good lubrication for the lower-temperature regions of a turbine engine, like the compressor section, he explains, and ceramic oxides or superalloys mixed with hexagonal boron nitride (
see Figure 6) work better in the combustion section, where temperatures are higher.
Figure 6. Ceramic oxides or superalloys mixed with hexagonal boron nitride (shown here) provide wear protection at high temperatures. Figure courtesy of Benjah-bmm27.
Solid coatings also protect the foil bearings in the small, high-speed turbocompressors used to recycle air within an aircraft cabin, Lince adds. The foil bearings (so named because of the spring-loaded foil journal liners) use air as the lubricating medium. At full operating speed, air is sufficient to keep the bearing surfaces separated, but coatings like PTFE protect the parts as the compressor ramps up from a stationary state or slows down after the motor is turned off.
Aircraft turbofan engines also use foil bearings, but the demands on these bearings, which can exceed 10,000 RPM and operate at much higher temperatures, are more severe. NASA has developed a series of solid lubricant coatings for high-temperature foil-air bearings, Lince says, with the latest being a plasma-sprayed material called PS400. This material is made from a nickel-molybdenumaluminum superalloy binder mixed with a chromium oxide hardener, a silver medium-temperature lubricant and a barium-calcium fluoride eutectic compound for lubrication at high temperatures. It forms dense coatings with a smooth surface finish and good oxidative and dimensional stability.
Argibay’s group is working on materials, including high-entropy alloys, that work well in high-temperature applications, including gas turbines and solar power plants that rely on molten salts and supercritical CO
2 as heat transfer and working fluids. “Most power plants tend to rely on some kind of heating of a fluid medium, like air or supercritical CO
2, and then feeding that hot and compressed gas through a turbine and producing power,” he says. “There are many ways to heat [this fluid], and that’s where the different fuels or power supplies, sources of energy, come into play.”
High-entropy alloys could allow these systems to operate at much higher temperatures than currently achievable. However, the alloy compositions need to function successfully when they are produced at a manufacturing scale, often a difficult or insurmountable obstacle for tech transfer and commercialization of new technologies. At the lab scale, Argibay says, you’re looking for tunability, exploring compositions and ranges of properties for the purpose of materials discovery. At the manufacturing scale, you’re looking for a reliable formula that gives you the same result every time. His group is currently looking at a new synthesis method for platinum-gold alloys that is highly scalable and cost effective.
Complex formulations provide versatility
Combining solid lubricants can produce complementary or synergistic effects (
see Figure 7). For example, Sumant’s group solution-cast graphene and MoS
2 onto a rough steel surface to form patchy areas of graphene and amorphous carbon (formed by the reaction of MoS
2 with graphene). This patchy coating reduces friction and wear, even at high contact pressures and sliding speeds.
10
Figure 7. Single-phase and composite materials provide various degrees of friction reduction and wear protection. Figure courtesy of Nicolas Argibay, Sandia National Laboratories.
Diamond-like nanocomposites (DLNs) use a mixture of hydrogenated amorphous carbon and amorphous oxygenated silicon. “You get the best of both worlds, since the carbon-hydrogen component gives you the harder diamond-like carbon properties. The amorphous silicon-oxygen component gives you lower friction and lower film stress,” Lince says. The addition of the solid silicon-oxygen phase helps the low-hydrogen DLC formulations commonly used for terrestrial applications to perform in dry environments, he says. This type of composite is used in various engines, printers and photocopiers, and its biocompatibility is being evaluated for use in coronary stents. Coating formulators often add a small amount of metal (e.g., tungsten, titanium or chromium) to DLN coatings to prevent the buildup of static charges, Lince says.
Combining carbon and larger amounts of metals (e.g., tungsten, titanium or chromium) forms composites containing a metal carbide phase for hardness and toughness and an amorphous carbon phase providing friction reduction capabilities. One common commercialized coating, tungsten carbide–carbon (WC/C) can be made with composition gradients that vary from the substrate surface to the interface. These hard, tough coatings are used in industrial machinery, car engine parts and ball bearings, where they can withstand high contact stresses and multiple cycles. WC/C coatings can react with the sulfur additives in a liquid lubricant to form a tungsten disulfide solid lubricant layer, Lince says, which works well under high loads and low speeds.
Conventional lubricants are largely formulated for use with steel, Lince says, but some of the additives they contain interact in interesting ways with metal surfaces. For example, molybdenum dithiocarbamate (MoDTC) contains sulfur, which can form MoS
2 under friction. The chemical underpinnings of these reactions have been studied for steel, and to some extent, aluminum and titanium alloys, but “when you start talking about these new solids, that’s really where there needs to be more work,” Lince says. “Steel was it for a long time. And now every time you introduce a new coating, the question is, is that going to work with our existing additives? Or do we need to come up with new ones? Some additives that don’t work well with steel do work well with some of these newer coating materials.”
Chameleon coatings are a kind of nanocomposite, Lince says, but they have their basis in macroscopic materials that have been used for decades. For example, MoS
2 and graphite powders, with a binder material like a resin, were painted onto the radomes of AWACS planes (
see Figure 8). The graphite reduced friction in moist environments like those near ground level, and the MoS
2 came into play in the dry environments at higher altitudes.
Figure 8. An AWACS plane radome (black disc at upper left), coated with a mixture of graphite and MoS2 to reduce friction on the ground and in the air. Figure courtesy of Tech. Sgt. Fernando Serna, U.S. Dept. of Defense.
Composite coatings can contain chromium nitride or titanium nitride phases that form sub-stoichiometric oxides (Ti
4O
7 or Ti
5O
9, for example) on their surfaces at about 700 C. These oxides, called Magnéli phases, contain atomic vacancies that form low-shear-strength planes that act as lubricants, Lince says. Hard composite coatings can be made with elemental metals like silver, which diffuses to the surface and provides lubrication at moderately high temperatures and oxides that form Magnéli phases at higher temperatures.
Designing coatings for use in multiple environments adds to this complexity. Some chameleon nanocomposites, still in the research phase, might contain gold (a moderate-temperature lubricant), a hard material like yttria-stabilized zirconia (YSZ), MoS
2 for low-temperature dry environments and DLC for hardness and low-temperature moist-environment lubrication, Lince says. The phases that work best in each environment automatically self-segregate to the surface under friction.
One complex nanocomposite coating made from YSZ-Ag-Mo was evaluated for use at temperatures up to 700 C. The silver provided lubrication below 500 C, while the Mo oxidized to form MoO
3 or a MoO
3-x Magnéli phase above 500 C. Success over multiple temperature cycles was elusive, Lince says, as the silver migrated to the surface too quickly and was lost. To remedy this, a diffusion layer (e.g., porous titanium nitride) can control the amount of silver that diffuses to the surface. These materials are still in the research phase, but significant progress is being made, he adds.
Coatings and their environment
Typically, engineers view environmental exposure as a problem to be solved, Argibay says, but certain combinations of materials and contact conditions can actually benefit from their target operating environment. Graphite lubricants, which require environmental water to be effective, are one classic example. “Anti-fragile” materials—such as metals that spontaneously form protective oxide coatings when exposed to air—can actually improve their wear resistance from a certain amount of damage, Argibay says. “Our team and the tribology community are finding increasingly clever ways to control certain aspects of the application, the material and the environment to try to generate self-correcting or improving conditions for the system—it’s a system-level response.”
Self-generating solid lubricants, currently in the research phase, represent a major change on the horizon in the lubrication industry, Sumant says. Some lubricant systems that undergo tribochemical reactions are essentially self-generating, “so there is an infinite supply of the lubricant, right there,” he says. “You don’t have to replenish it because a tiny fraction of this will produce millions of nanocarbon [particles].”
Recently, Argibay and his colleagues made the serendipitous discovery that even in an inert atmosphere, a shear-assisted reaction transforms trace amounts of organic compounds on a highly wear-resistant nanocrystalline platinum-based alloy coating into a form of superlubricious tribofilm. This tribofilm is even more wear-resistant than the alloy coating, with tribological properties resembling those of engineered DLC coatings.
11 His group found that under a nitrogen atmosphere containing as little as parts-per-billion concentrations of organic contaminants, a sliding contact between an alumina sphere and an Pt-based alloy-coated steel surface achieved a coefficient of friction as low as 0.01.
Singh also has done research on material combinations that form tribofilms under shear. For example, MoS
2 added to a base oil acts as a catalyst to form a tribofilm,
12 and some DLC systems also form tribofilms under shear. This is a cleaner way of making
in situ films and environmentally friendly lubricants, he says, noting the absence of hazardous additives or decomposition products in these systems. Singh and his colleagues at Argonne National Laboratory studied a highly hydrogenated DLC material in air for potential use with wind turbines.
13 This material, used with a lubricant under mixed rolling and sliding contact, forms protective, self-replenishing hydrocarbon tribofilms on steel surfaces, preventing micropitting of the sort observed on wind turbine bearings.
Return on investment
Singh notes that economical, mass-produced solid coatings, including graphene-based or superlubricating coatings, could save companies billions of dollars by improving overall efficiency and reducing the overall sizes of mechanical systems. Generally, mechanical parts must be made to a certain minimum size to provide them with the strength they need to withstand friction (and the heat it generates), loads and shear forces. Thin solid coatings, just few atoms thick, that provide ultra-low friction could allow smaller parts to stand up under typical operating conditions, providing the same or better performance.
Even the solid coatings that are already on the market can withstand millions of cycles under typical operating conditions before they wear through to the metal underneath, Singh says. This can represent several years of operation before the coating or the part has to be replaced.
REFERENCES
1. Sliney, H.E. (April 1991), “Solid Lubricants, NASA Technical Memorandum 103803.” Available at
here.
2. Paul, A., et al. (May 16, 2018), “An Improved Solid Lubricant for Bearings Operating in Space and Terrestrial Environments,” NASA/CP—2018-219887. Available
here.
3. McGuire, N. (2020), “Solid lubricants for the aerospace industry, TLT,
76 (8), pp. 30-36. Available
here.
4. Berman, D., et al. (2014), “Graphene: a new emerging lubricant,”
Materials Today, 17 (1), pp. 31-42.
5. Berman, D., et al. (2015), “Macroscale superlubricity enabled by graphene nanoscroll formation,”
Science, 348 (6239), pp. 1118-1122.
6. Berman, D., et al. (2018), “Operando tribochemical formation of onion-like carbon leads to macroscale superlubricity,”
Nature Communications, 9: 1164, Doi: 10.1038/s41467-018-03549-6.
7. Sumant, A.V. and Berman, D. (August 18, 2020), “Low friction wear resistant graphene films,” U.S. Patent 10,745,641 B2.
8. Holliday, R. and Goodman, P. (May 2002), “Going for gold [gold in electronics industry],” in
IEE Review, 48 (3), pp. 15-19, Doi: 10.1049/ir:20020302.
9. Chou, E. (July 4, 2018), “Gold and the electronics sector,” Goldhub. Available
here.
10. Mutyala, K. C., et al. (2019), “Graphene - MoS
2 ensembles to reduce friction and wear in DLC-Steel contacts, Carbon, 146, pp. 524-527. Available
here.
11. Argibay, N., et al. (2018), “
In-situ tribochemical formation of self-lubricating diamond-like carbon films,”
Carbon, 138, pp. 61-68.
12. Mutyala, K. C., et al. (2016), “Influence of MoS
2 on the Rolling Contact Performance of Bearing Steels in Boundary Lubrication: A Different Approach,”
Tribology Letters, 61, Article No. 20.
13. Singh, H., et al. (2016), “Fatigue resistant carbon coatings for rolling/sliding contacts,”
Tribology International, 98, pp. 172-178.