Solid lubricants for the aerospace industry
Dr. Nancy McGuire, Contributing Editor | TLT Solid Lubricants August 2020
Planning and investing in coating formulations, application methods and testing help ensure that satellites and landing craft hold up for the life of the mission.
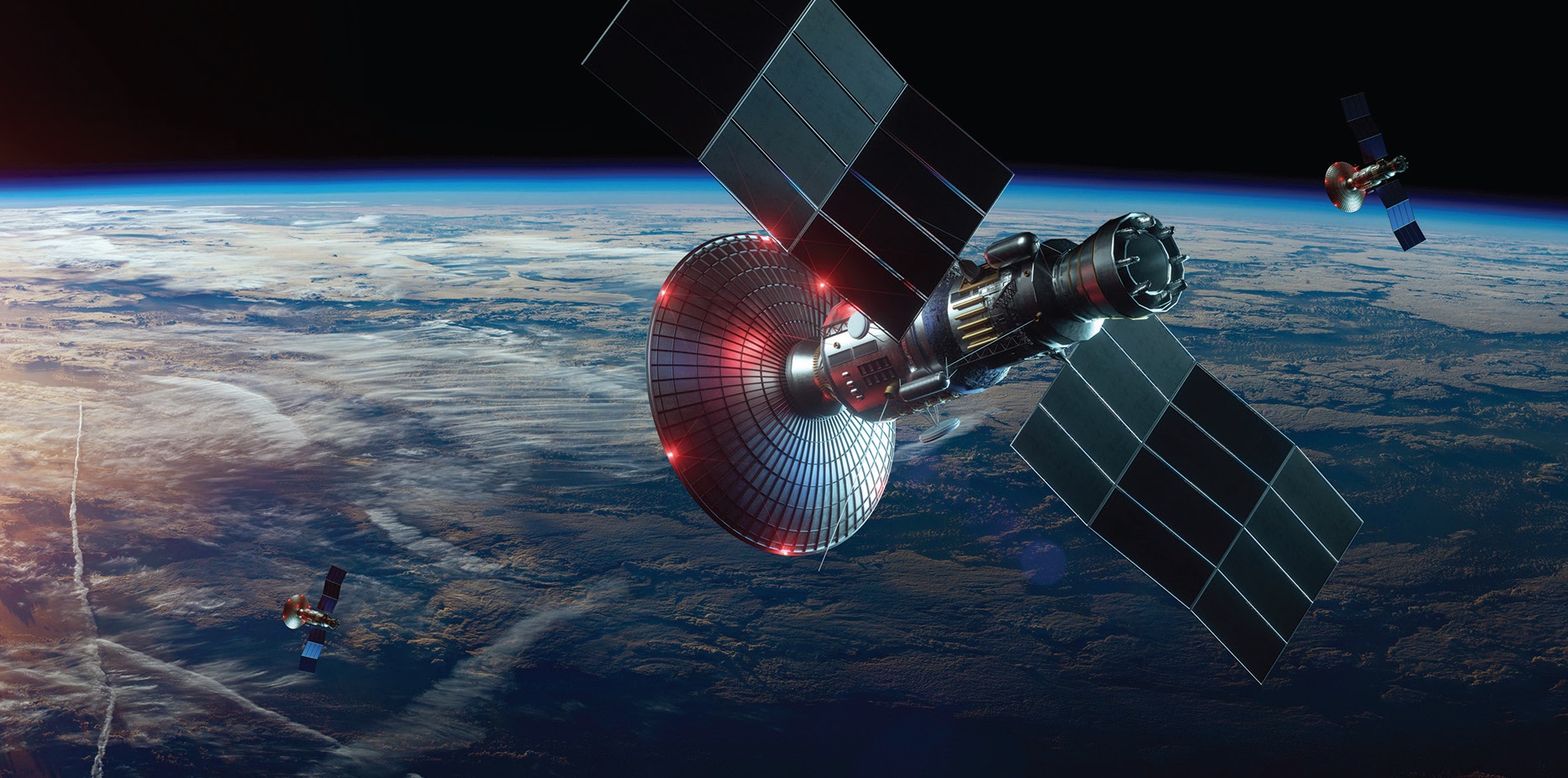
Satellites orbiting Earth must survive launch conditions, cleanly deploy solar panels and antennas and survive extreme temperatures and cosmic radiation. Image furnished by NASA.
KEY CONCEPTS
•
Including solid lubricants in the system design process from the very beginning increases their chance of success.
•
Engineering models should be tested in an environment that resembles actual operating conditions as closely as possible.
•
Using a less expensive coating might save a small fraction of a satellite’s cost, but if it fails, it can jeopardize an entire mission.
Spacecraft systems might be many thousands of miles from the nearest repair technician, so the mechanical parts must operate as intended for the duration of the mission. “If it doesn’t work, that’s the end of the mission, so it has to release very cleanly,” says STLE-member Dr. Jeff Lince, principal at Space Tribology Consulting in Culver City, Calif., referring to satellite release and deployment mechanisms. These mechanisms must hold their sensors, antennas and solar arrays very securely during launch and then release them smoothly and accurately when the satellite reaches orbit. Thus, the success of an entire mission can rest on a thin film of solid lubricant.
Lince helps his clients develop effective means of using tribological materials, including solid lubricants, for spacecraft and other systems that operate in a vacuum environment. The word “clean,” he notes, refers not only to smooth mechanical operation but also an operation that does not release contaminants onto the devices being deployed. Ideally, solid lubricants stay on the surfaces where they are put, even when those surfaces are exposed to the vacuum and microgravity of space, where liquid lubricants could evaporate or migrate along surfaces outside of the mechanical contact zone, contaminating sensors or other spacecraft parts with a lubricant film.
Solid lubricants also function at extreme temperatures where the viscosity of oils and greases is too high or low to be effective. These lubricants are used in cryogenic liquid hydrogen–oxygen rocket fuel systems. Turbopumps force the pressurized liquid fuel components into the engine where an electrical spark ignites them. Finding a lubricant that will work with the pump bearings is challenging, Lince says. Liquid lubricants will freeze, and hydrocarbon lubricants can explode in this type of liquid fuel environment. Thus, the bearing cages and retainers use solid materials that form lubricating transfer films to the rings and balls that they slide against. After the turbopump bearings in the main engine of the Space Shuttle experienced wear problems, for example, they were replaced with upgraded bearings with ball cages made of a bronze/PTFE solid lubricant, silicon nitride balls and 440C steel races (
see Figure 1).
1
Figure 1. Upgraded turbopump bearings in the main engine of the Space Shuttle used ball cages made from a bronze/PTFE lubricant material, silicon nitride balls and 440C steel races. Figure courtesy of Jukka/Flickr, CC BY 2.0.
Slip ring assemblies, devices that enable electrical signals and currents to pass through a rotating interface, are another area for solid lubricants. Here, materials must not only reduce friction and wear, but they must also be electrically conductive, which is sometimes mutually exclusive, Lince says. Mercury is sometimes used for this purpose here on Earth, but mercury’s vapor pressure is too high for it to be practical in space. One solution, he says, is to mix solid lubricants with conductive metals to strike a balance between good tribology and high electrical conductivity.
Getting things right from the start
“What some people sometimes erroneously do,” Lince says, “is they design their mechanism, then the subsystem that the mechanism fits into and then the satellite…and then they say, ‘Well, what kind of lubricants should we use?’” Often, they use whatever worked for them last time, he continues, but if the part geometry, materials or loads are even slightly different, that might be enough to warrant a different lubrication scheme. “That’s a big problem,” he says.
Solving the problem requires identifying the right solid lubricant, Lince continues. He notes that ensuring success requires folding several interacting factors into the design from the very beginning, “before your preliminary design review,” so that the system works, even under circumstances that weren’t predicted.
Getting it right involves choosing the right substrate materials—for instance, chemically dissimilar materials that will not gall if they come into contact during operation. Surface treatments make surfaces more chemically resistant, which also protects them if the lubricant fails and the surfaces come into contact. The environment—or often, environments—of the lubricated part also are critical considerations. Before a lubricant and the mechanical system it protects encounter the cold and vacuum of outer space, they can be exposed to Earth’s humid, oxidizing atmosphere during assembly, packaging and testing, followed by heat and vibration during transportation and launch (
see Kennedy, We Have a Problem). Lince notes that systems must be designed with safety margins that greatly exceed the expected operating conditions.
Solving lubrication problems early in the design process is one thing that Dr. Malcolm Stanford likes about working at NASA. Stanford, a materials research engineer at the NASA Glenn Research Center in Cleveland, Ohio, specializes in materials development for friction and wear reduction in aeronautics and space applications. In his previous job in private industry, the problems were driven by more immediate deadlines. Even at NASA, Stanford says, deadline pressure can come into play when there’s a specific issue with something that’s out in orbit already, especially if there’s a crew involved or if an issue comes up and there’s a launch date coming up soon. “The bulk of my work, however, is to look for solutions to problems that are a little further out.”
“At Glenn, we form teams that usually interact over telecoms,” Stanford says. “The flight hardware community has a set of parameters that determines what our envelope is, and that is what the researchers in the materials lab have to work with.” Sometimes during the course of a project, the parameters or conditions change. Team members work back and forth to come up with tradeoffs in making one design choice or another. A typical envelope of operating conditions is defined by such things as the atmosphere in which the system operates, the pressure, the speeds and sometimes the counterface material.
Hostile destinations
Landing craft for planetary missions present their own set of challenges. Landing craft and instruments must be protected from Earth’s oxidizing atmosphere, but they also must be able to function in the chemical and physical environments of their destination sites.
“Satellites sitting in orbit have just a few things turning around, and for the most part, they turn around the same way every day,” Lince says. “But when you have rovers on a planet’s surface, extremely complex pieces of equipment that can be the size of a Mini Cooper, they have to move around in extremely dirty, dusty, abrasive environments with temperature extremes. Windstorms that coat your camera lenses and things like that. And you also have to manipulate small amounts of samples and do science experiments on them.”
Stanford is part of a research effort to enable long-lived probes to function in the ambient environment on the surface of Venus. In the very hot, high-pressure atmosphere of Venus, CO₂ behaves more like a liquid than a gas, and corrosive acids in the atmosphere could make short work of delicate instruments. “This is everything that an extreme environments tribologist dreams of,” he says.
“We have a Venus simulation chamber that allows us to expose materials to that environment and see what will survive in that atmosphere,” he continues. “We have to know how our lubricants will behave in a relevant environment, so the solid lubricants that we use for the vacuum of space are not necessarily the ones that are going to work in an environment like that on Venus.” He adds that, although the surface probe would be traveling through space on the journey to Venus, it would not be designed to work in that cold vacuum. Rather, it would be stowed aboard the spacecraft and then deployed in the environment it was designed for.
The Mars Curiosity rover’s many mechanical parts function despite temperature extremes and dirty, dusty, abrasive environments such as windstorms. Image provided by NASA.
Solid lubrication and wear protection also can protect mechanical parts in dry or dusty environments like those on the Moon or Mars. This isn’t a problem for some components, like gears and bearings that are sealed off from the environment, Stanford explains. However, the Apollo lunar rovers had issues with dust contamination, and the aluminum wheels on the Mars Curiosity rover started to wear more quickly than anticipated because of the hard, rocky environment it was rolling over. Over the years, NASA has developed a variety of surface rover wheel designs in an effort to make a wheel that provides enough traction but lasts long enough to work for the duration of the mission (
see Figure 2).
Figure 2. After a few months of rolling over the rocky surface of Mars, the aluminum wheels on the Mars Curiosity rover showed wear marks and holes. Figure courtesy of NASA/JPL-Caltech/MSSS.
Lince has been involved in the upcoming Mars 2020 mission, where the sample collection system has literally thousands of moving parts. Many of these have to be lubricated with solids because liquid lubricants will contaminate the samples. “Every new mission to a planet has some new thing that they’re doing. And I think that we’re going to have to come up with new tribological materials in order to meet their requirements for these difficult new missions,” he says.
Applications closer to home
Satellites orbiting the Earth face their own challenges, including exposure to chemically active high-atmosphere species like atomic oxygen. With less (or none) of Earth’s atmosphere to protect them, these satellites also are exposed to high-energy solar radiation and thermal extremes, as well as the effects of microgravity and vacuum.
Here on Earth’s surface, medical instruments, metalworking tools, seals, hydraulic fittings and vacuum-enclosed instrumentation also might require solid lubricants. High loads, low speeds, high radiation levels and sensitivity to contamination are a few of the operating conditions and environments where it is not feasible to use oils and greases. For example, environments in the ITER (originally the International Thermonuclear Experimental Reactor) combine cryogenic temperatures, high radiation levels and high vacuum. Solid lubricants are critical to this multibillion-dollar international project, aimed at building a nuclear fusion reactor, says STLE-member Dr. Brandon Krick, associate professor of mechanical engineering at Florida State University in Tallahassee, Fla.
Molybdenum disulfide (MoS
2) and PTFE, two solid lubricants that are well established in aerospace applications, perform best in a vacuum. MoS
2 is sensitive to moist air environments, so storage and testing conditions must be carefully controlled, but a variety of available formulations can address this type of performance issue. For example, MoS
2 mixed with a resin binder can be painted onto a surface to form a bonded coating. The way that any particular type of coating is applied will have a significant effect on the composition and its sensitivity to the environment, Lince explains, noting that MoS
2 also can be applied using physical vapor deposition. “With careful understanding of the material and chemical properties of the formulation you’re using, you can tailor the coating so that you can test it on Earth in humid air for short periods and then launch, and then it still works once it’s gotten up to space.”
Aircraft turbine engines operate most efficiently when clearance between the turbine blades and the engine housing is very small to minimize the amount of gases escaping.
“There are hundreds if not thousands of formulations of MoS
2 out there,” Lince says, because it’s used for terrestrial applications as well as aerospace. For example, sputter-deposited adaptive chameleon coatings combine MoS
2 for performance in vacuum and graphite for performance in ambient air. Other species can be added to enhance high temperature performance. Hard nanocomposite films made from co-sputtered metals and MoS
2 are used for applications involving high contact stress. Other combinations (e.g., gold co-sputtered with 11%-25% MoS
2) outperform either component alone under the low contact stresses typical of electrical slip rings. The metal component provides hardness, fracture toughness and resistance to oxidation in air, while the MoS
2 component concentrates in the surface region to provide lubrication.
2
Aircraft turbine engines, another major area of application, operate most efficiently when the clearance between the turbine blades and the engine housing is very small to minimize the amount of gases escaping around the edges. The blades can actually touch the housing, which requires the use of sacrificial coatings to prevent turbine blade wear, Lince says. Superalloys (e.g., Inconel and Hastelloy) made from various combinations of nickel, iron, cobalt and other elements are strong like steel but stand up better at the elevated temperatures typical of the turbine’s interior surfaces. Wear protection alloy materials are mixed in with solid lubricants to form composites. Graphite can be used to lubricate lower-temperature areas like the inlet stage, and boron nitride can be used for the high-temperature areas, he says.
Invest now, save later
The cost of coating materials is actually a small part of the total cost of a space mission, Lince says, although it might require a significant investment of time to test the wear protection coatings and solid lubricants. Tribologists use a variety of standard tests (e.g., pin-on-disk) to screen lubricants, but often the laboratory test does not model the actual application very closely, especially when the application is in space. In situations where standard tests are difficult to perform in a vacuum, lubricant screening can be done under a dry argon or nitrogen atmosphere.
Engineering models should be tested in an environment that resembles actual operating conditions as closely as possible—“test like you fly,” Lince says. “It’s very important, especially if it’s a new design and a new material. You want to make sure that it’s going to work once it gets up into space, so you have to test it in as close to flight-like conditions as possible.” Researchers from the University of Florida took this mandate quite literally about 10 years ago, when they built eight tribometers for testing materials in low Earth orbit on the International Space Station.
3
Often, Lince says, testing like you fly means testing the device in a vacuum chamber, which might require finding a way to provide electrical power or other connections to the device inside the chamber. “It can be very difficult,” he says. “But then a little bit of difficulty in setting up accurate testing can save you a lot of heartache later on if you discover the problem late in the whole manufacturing cycle and you’ve got to backtrack—or even worse if you don’t have a problem until it gets into orbit.”
Long-term thinking also pays off for cost analysis. Lince explains that sputter-deposited coatings based on MoS
2 can be somewhat expensive because they require application using plasma technology in a vacuum chamber. Bonded coatings, which can be sprayed or brushed onto a surface and baked on in an oven, are generally less expensive. However, in many applications, the sputter-deposited coating might be a better investment because it is highly adherent, provides low friction and lasts a long time. Sputter-deposited coatings can be thin enough to avoid significantly changing the coated part’s dimensions, which is important for mechanisms like ball bearings that have small tolerances. Lince notes that if a satellite costs hundreds of millions of dollars, saving a few thousand dollars by using a coating that is not suited to the application might be a false economy, especially if a coating failure shortens the life of the satellite.
A component failure before launching a satellite or planetary exploration mission is expensive and frustrating, Lince says. However, he adds, a failure after launch can cause loss of mission, which would have serious implications for national security, e-commerce, satellite navigation systems or communications networks. Dead satellites and other spacecraft debris in low Earth orbit also pose a hazard to functioning satellites.4 Thus, it pays to invest up front in coatings, lubricant formulations, application methods and handling conditions that ensure a given system will hold up for the life of the mission.
REFERENCES
1. Gibson, H. (August 2001), “Lubrication of Space Shuttle Main Engine Turbopump Bearings,”
Lubrication Engineering, pp. 10-12.
2. Lince, J. R. (2004), “Tribology of co-sputtered nanocomposite Au/MoS
2 solid lubricant films over a wide contact stress range,”
Tribology Letters,
17 (3), pp. 419-428.
3. Krick, B. A. and Sawyer, W. G. (2011), “Space Tribometers: Design for Exposed Experiments on Orbit,”
Tribology Letters,
41, pp. 303-311. Available
here.
4. European Space Agency (February 2020),
Space Debris by the Numbers. Available
here.
In December 1995, Galileo became the first spacecraft to establish an orbit around Jupiter. Artist’s conception courtesy of NASA/JPL.
Kennedy, we have a problem
In April 1991, NASA’s Galileo satellite failed to fully deploy its 16-foot-diameter high-gain antenna (
see illustration). The antenna was designed to unfold like an umbrella after Galileo established its orbit around Jupiter, but several of its 18 ribs stuck in the closed position.
Galileo’s antenna problems started before it was ever launched. The antenna was built in Florida, shipped 2,500 miles to the Jet Propulsion Laboratory (JPL) in California for testing and shipped back to Florida’s NASA Kennedy Space Center for Galileo’s scheduled launch in May 1986. After the Challenger disaster in January 1986, Galileo and its antenna were shipped back to JPL. The spacecraft was shipped back to the Kennedy Space Center for a successful launch in October 1989. A 1999 study noted that by the time the antenna was launched into space, it had rattled over 10,000 miles worth of public highways.
A
A 1994 postmortem report concluded that high contact stresses on V-groove pin–socket interfaces destroyed the integrity of the solid lubricant film on the pins, causing cold welding between the pins and sockets. Although conical pins and sockets were exposed to the same environmental factors, they experienced different levels of contact stress and did not fail.
B
Much of Galileo’s prelaunch testing was done in Earth’s ambient atmosphere. In-air testing of parts coated with a MoS
2 dry lubricant might have contributed to their failure because the wear rate of MoS
2 is much higher in air than in a vacuum. The titanium pins readily formed an oxide coating in air, so in-air testing did not detect the change in friction caused by the damaged surfaces. A functional test of the flight antenna performed under vacuum did not include the same degree of relative motion between the pins and sockets, and thus did not exhibit this failure mode. “The test conditions were not adequate for finding this problem, indicating that just a functional test in vacuum is not always appropriate,” the postmortem report concluded.
B
REFERENCES
A. Miyoshi, K. (1999), “Aerospace Mechanisms and Tribology Technology: Case Study,” NASA Technical Memorandum NASA-TM-1999-209077. Available
here.
B. Johnson, M. R. (1994), “The Galileo High Gain Antenna Deployment Anomaly,” NASA Technical Report 19940028813, pp. 359-377, NASA-CP-3260. Available
here.