Formulation challenges for industrial gear lubricants
Dr. Nancy McGuire, Contributing Editor | TLT Webinars September 2018
Moisture, temperature, viscosity, filtration and base oil are just some of the factors you’ll need to consider.
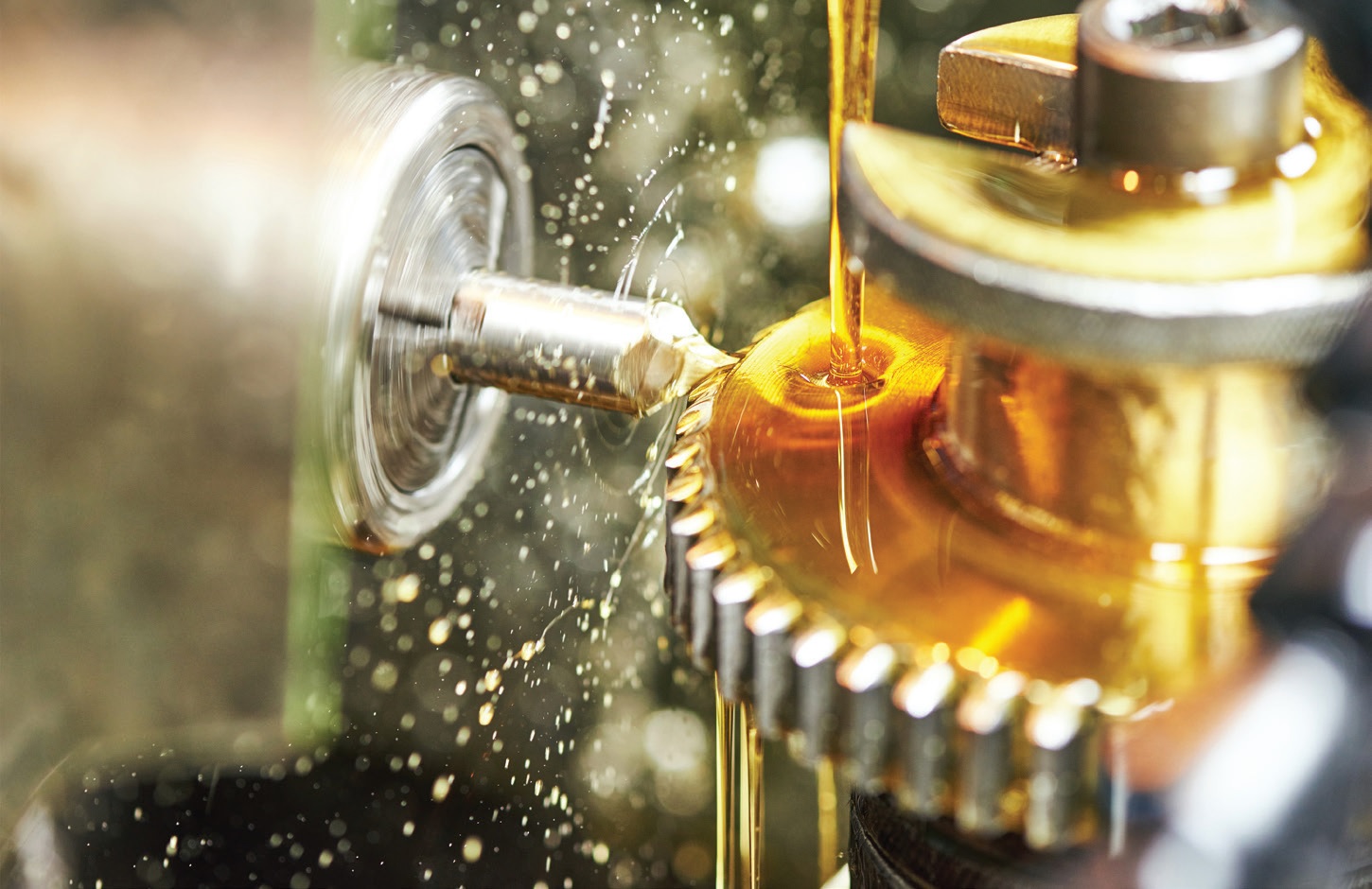
© Can Stock Photo / kadmy
KEY CONCEPTS
•
Gear oils must be formulated to fit specific gear speeds, loads and operating conditions.
•
Newer equipment requires less lubricant, but the lubricant must operate at higher temperatures and power densities.
•
Manufacturers’ recommendations are the best guide to selecting an industrial gear oil.
MEET THE PRESENTER
This article is based on a Webinar originally presented by STLE Education on Oct. 18, 2017.
Challenges to Formulating and Selecting Gear Lubricants and Their Applications is available at
www.stle.org: $39 to STLE members, $59 for all others.
Lawrence Ludwig, Jr., is chief chemist/technical director for Schaeffer Manufacturing Co. in St. Louis, Mo., where he has been employed for 40 years. He is an active member of STLE, has chaired several technical committees and served in local section positions for the society’s former St. Louis Section.
He is a member of ASTM, ASME and SAE, as well as a member of the NSF International’s Non-Food Compounds Registration Steering Subcommittee and NLGI’s Food Grade Lubricants and Bio-based Lubricants Working Groups. Ludwig graduated from St. Louis University in 1977 with a bachelor’s of science degree in chemistry. He is one of a few people in the world holding STLE’s Certified Lubrication Specialist™ (CLS), Certified Oil Monitoring Analyst I™ (OMA I) and Certified Metalworking Fluids Specialist™ (CMFS) certifications. You can reach Ludwig at
lludwig@schaefferoil.com.
Lawrence Ludwig, Jr.
Gears transmit motion from one shaft to another or from a rotating shaft to a reciprocating element. Gears transmit power, change speed, change force (torque) or change direction. They operate under a myriad of operating cycles, loads, speeds and temperatures (
see Gear Basic Terminology).
Gear basic terminology
Clearance is the distance between the top of one tooth on one gear to the base of the tooth on the other gear (the difference between the dedendum for one gear and the addendum of its mating gear). Proper clearance allows the gears to mesh properly and provides sufficient space for the lubricant to penetrate and perform properly.
Pitch is the distance between a pitch point on one tooth and the corresponding point on an adjacent tooth where the gears come into contact. High-speed gears have a small pitch and short teeth to reduce sliding; low-speed, high-load gears have large teeth to reduce tooth breakage.
Backlash, the distance between the back of one tooth and the front of the next mating tooth, is critical to allow lubricants to coat the teeth properly and prevent overheating, noise, tooth wear and tooth failure.
(Illustrations courtesy of Schaeffer Manufacturing Co.)
Gear lubricants increase the durability of equipment by preventing gear and bearing wear and preventing excess friction. Lubricants also carry heat generated by equipment away from the contact area. Gear lubricants can enhance the cleanliness of gears, bearings and seals by preventing rust, corrosion and other types of deposits from forming. They also can prevent air from being entrained in the lubricant and retard foaming of the lubricant. A properly functioning lubricant reduces power consumption and noise.
Challenges and trends
Today’s customers demand that equipment be more durable and reliable with longer drain intervals. Industrial gearboxes use new materials and surface finishes to provide more power with less weight, size and manufacturing costs (
see Figure 1). However, modern gearboxes run hotter and, with less lubricant to cool the system, these gears experience increased thermal stress and higher loads on gear teeth and bearings.
Figure 1. Gear drives are as much as five times smaller and lighter than they were 50 years ago. (Figure courtesy of Schaeffer Manufacturing Co.)
Challenges to gear operation include the presence of large quantities of water or moisture, high operating and ambient temperatures or environments that are highly contaminated with chemical vapors, dust or dirt. Filtering lubricants can selectively remove some lubricant additives, and this must be dealt with accordingly. Fluctuations in speed and loading may cause speeds and loads to intermittently exceed the ratings for the gear. Certain gear types and applications require protection from micropitting.
Trends
Baseline performance requirements for gear lubricants are constantly changing. Today’s gear lubricants must be stable against oxidation, viscosity changes and thermal degradation under more stringent conditions. OEMs and customers require gear lubricants that provide increased thermal stability, cleanliness and wear protection at higher power densities and operating temperatures. Other requirements include gear and bearing durability for extended service life, materials compatibility, improved energy efficiency, use of sustainable additives and base oils, extended drain intervals and lower cost.
Factors that affect gear lubrication performance include the type of gear (
see Figure 2), its surface finish (rougher finishes require higher viscosity lubricants), its speed (higher speeds require lower viscosities), loading and transmitted power (heavy loads require higher viscosity), ambient and operating temperatures, materials compatibility, contamination, application methods (splash or circulation), filtration and operating conditions.
Figure 2. Top row, left to right: Spur, helical and spur or straight bevel gears. Bottom row, left to right: Worm and herringbone gears. (Figure courtesy of Schaeffer Manufacturing Co.)
A gear lubricant must have the correct viscosity at the operating temperature to distribute lubricant to all contact surfaces and form an elastohydrodynamic lubrication (EHL) film, but it also must exhibit adequate fluidity at low temperatures, especially at startup. It must be chemically stable, show good demulsibility characteristics (expulsion of water) and provide extreme pressure (EP) and antiwear (AW) protection.
Several standards for classifying and providing specifications for gear lubricants ensure that each type of lubricant meets the minimum performance characteristics that OEMs require for their products and applications. The most-referenced specifications are AGMA 9005-E02, AGMA 9005 F-16 (the most current specification), and DIN 51517, which has parts for rust and oxidation (R&O), R&O antiwear and EP-type gear oils. Some OEMs are now requiring thermal oxidation stability tests such as the L-60-1 Thermal and Oxidative Stability Test ASTM D-5704 more frequently in their specifications for industrial gear lubricants.
Base oil selection
Selection of a base oil depends not only on the equipment’s operating conditions (temperatures, loads and susceptibility to contamination) but also the types and amounts of additives that go into the formulation, as well as the types of gear, bearing and seal materials with which the lubricant will be interacting. Biodegradability of the base oil is another consideration, especially for operations that are done in an environmentally sensitive area. The cost of a lubricant involves not only the cost of the base oil itself but also the additive and production costs, any energy efficiency that the lubricant can provide and the drain intervals and service life of the lubricant.
The type of base oil has a significant effect on performance. Base oil choice affects overall base oil film thickness and viscosity which, in turn, affects film thickness in the hydrodynamic and elastohydrodynamic regimes. The properties of the base oil determine the compatibility and solubility of various additive systems in the base oil and the responses of these additives during use. Base oil choice also affects thermal and oxidative stability, rheology at low and high temperatures, hydrolytic stability, tendency toward foaming, heat transfer and compatibility with seals and other materials.
Petroleum-based (mineral oil) gear lubes use Group I and Group II base oils. Synthetic base oils include polyalphaolefins (PAO), esters, polyalkyline glycols (PAG) and Group III oils (with the use of polymers). Mineral and synthetic oils can be blended to form semi-synthetic (synthetic blend) bases.
Group I oils lack the antioxidant properties of Group II and Group III oils, but their performance is easily enhanced using additives. Group I oils hold additives better (solvency) than Groups II and III, have a lower cost and are available in a wider variety of viscosity cuts.
Group II and Group III oils provide better thermal and oxidative stability and can work synergistically with carefully chosen antioxidant additives. Because these oils have decreased solvency, additives must be selected carefully. These base oils are useful for higher-temperature lubricants, and they are only available in lower-viscosity cuts (ISO 68 to 100). These oils may be blended with PAOs, polymers or Group I oils to increase the viscosity of the lubricant. Although Group II and Group III base stocks tend to cost more than Group I base stocks, their increasing availability is bringing their price down.
Synthetic base oils perform very well for low-temperature operations, but they also provide better thermal and oxidative stability at high operating temperatures. Synthetics offer better heat transfer characteristics, lower friction, higher shear strength and lower coefficients of traction than do mineral oils.
Base oil choice affects the coefficient of traction which, in turn, influences the energy savings that a lubricant can achieve (
see Figure 3). The coefficient of traction is an indicator of a lubricant’s molecular resistance to shearing.
Figure 3. Traction is a lubricant’s molecular resistance to motion of one layer of fluid sliding over or along another layer of fluid. (Figure courtesy of Schaeffer Manufacturing Co.)
PAOs and PAGs have the lowest coefficients of traction, but synthetic oils can be at a disadvantage with respect to pour solubility for some additives. Esters and PAGs may exhibit compatibility problems with some jointing materials and seals, and silicones and PAGs may be immiscible in other base oil types. Certain types of esters may reduce the hydrolytic stability of a lubricant.
Additives
Lubricant additives serve a variety of functions. Some additives modify properties of the base oil—they inhibit oxidation, corrosion, foaming and water ingress. They also can act as pour point depressants and viscosity index improvers. Other additives—EP and AW additives, friction modifiers, detergents, dispersants, metal deactivators and tackiness agents—can provide new properties to the lubricant. All of these functions require mixtures of additive components, and the systems of interacting components can become very complex.
Frictional modifiers reduce friction and wear during boundary and near-boundary lubrication conditions. These compounds consist of long hydrocarbon chains with a small polar functional group at one end. The polar groups anchor themselves onto a metal surface, leaving the hydrocarbon tails facing toward the interfacial area. Aggregates of these molecules function as a tough, wear-resistant polymer film. Frictional modifiers can act as AW additives, EP additives and oxidation inhibitors.
EP lubricants protect geared systems that operate under high loads and severe impact or reversing conditions. EP agents react chemically with metal surfaces to form a film that protects against metal-to-metal contact and adhesion. This protective boundary lubricant film fills in surface asperities to reduce friction, welding, scuffing and scoring under high-speed/high-load conditions, low-speed/high-shock-loading conditions or high-temperature conditions. Typical EP agents include sulfur-phosphorus, borates and sulfur-phosphorus-boron.
Antiwear additives reduce friction and wear under mixed-film and boundary lubrication conditions by preventing metal-to-metal contact. These additives prevent galling, scoring and seizure of critical moving parts. Antiwear additives form films on the surface either through physical adsorption or by chemically reacting with the metal surface to form a low-shear film at the point of contact.
Solid lubricants reduce friction and wear by forming thin solid films between rubbing and sliding surfaces. These lubricants remain in place even when high loads and pressures squeeze out liquid lubricants.
Rust and oxidation (R&O) inhibited lubricants are generally associated with applications requiring higher gear speeds and lighter loads. Rust inhibitors protect ferrous metal surfaces from oxidation, and corrosion inhibitors protect nonferrous metals from attack by acids. These additive compounds commonly have a polar functional group that attaches to the metal surface and a long hydrocarbon tail that extends into the interface and repels water or acids. Over-based detergent molecules also can neutralize acids, and dispersant compounds can surround water or acid droplets, preventing the droplets from coming into contact with the metal surface.
Antioxidants prevent the formation of acids, gums, resins, varnish and sludge that normally results from the process of oxidation. These additives can extend the life of a lubricant by a factor of 10-100 by scavenging free radicals or peroxides as they are formed during the oxidation process, preventing them from further reacting with the lubricant.
Demulsifiers promote the rapid separation of water from the oil; they enable small, stable water droplets to form bigger droplets that separate out and sink to the bottom of the oil reservoir where they can be drained. Demulsifiers have limited solubility in oil (typical formulations use only 200-400 ppm), and using too much can actually promote the formation of emulsions.
Anti-foam agents prevent the formation of stable foams and entrained air in a lubricant. These additives reduce the surface tension of oil-air droplets so that air can be quickly released from the oil. Removing air from the lubricant not only retards oxidation, but because air acts as an insulator, removing it helps the lubricant do a better job of channeling heat away from the gear system. Adding excessive amounts of anti-foam agents, however, can promote air entrainment, which may result in lubricant cavitation and gear pitting. Anti-foam agents have limited solubility in the oil, and filtration or other factors can separate them out from the lubricant.
Pour point depressants improve the flow properties of mineral base oils at low temperatures. These additives retard the formation of large needle-like aggregates of wax crystals by coating the surfaces of the crystals as they form, resulting in the formation of smaller, more rounded wax particles that do not retard the oil from flowing.
Viscosity modifiers (high molecular weight polymers) modify the way the oil’s viscosity changes with temperature. At low temperatures, the polymer molecules coil into small particles that offer little resistance to flow. At higher temperatures, the polymers uncoil to form fibers that restrict flow, preventing the viscosity from dropping too low. Viscosity modifiers must resist mechanical shear, and they must be chemically and thermally stable.
Additive interactions
Gear oil additives interact with each other, the base oil and the mechanical parts they contact. Additive solubility and compatibility, shear stability, materials compatibility and filtration must all be taken into account.
Balancing additive chemistry with the base oil can reduce or prevent compatibility problems with seals. Seals often fail when they harden or when deposits form on them, and these effects are especially pronounced at high operating temperatures. Deposits can form around the seal lip when thermal stress breaks down a gear lubricant. These deposits can abrade the seal material, causing cracking and tearing. Chemical interactions between the seal material and the lubricant can cause the seal to become less elastic and allow it to leak. Some types of elastomer seals can swell and plasticize when exposed to incompatible lubricants. PTFE seals (e.g., Teflon®) and Viton® seals provide the most universal compatibility.
Using appropriate lubricant additive chemistry can dramatically reduce micropitting, in which very small cracks and pores form on the surfaces of gear tooth flanks, causing the metal surface to appear gray, white or frosted. This problem is seen with wind turbines or other gear systems that undergo a lot of vibration or with case-hardened gears that have very smooth surfaces. Micropitting causes material loss and a change in the profile form of the tooth flanks, which can lead to pitting and changes in the gear tooth profile, and it concentrates the load over a smaller area.
Some types of EP additives contain active sulfur compounds that can corrode yellow metals. High operating temperatures can thermally stress some EP additives, producing radical changes in their chemical structure, leading to an increased risk of spalling, ridging, overall distress and increased deposit formation.
Today’s smaller gear drives use smaller volumes of lubricants. Although this is good from an economic standpoint, there is less time for contaminants and deposits to settle out in the reservoir before the oil comes back into contact with the mechanical parts. The longer the fluid is kept in the system, the more cleanliness becomes an issue. Filtration can help, but highly viscous oils may be difficult to filter. Heating the filter or the oil itself can help the oil to pass through the filter. Filtration may remove beneficial components of the lubricant, including tackiness agents, suspended-solid additives and anti-foaming agents.
Lubricant selection
Following the manufacturer’s recommendations is the best guide to selecting the proper lubricant for a piece of equipment. This information may be inscribed on name plates, included in equipment and owners’ manuals, or listed on the manufacturer’s Website. These specifications are designed to balance the various lubrication needs of the bearings and gears.
Viscosity is the most important property of any lubricating oil. Selecting an oil with the right viscosity for the application ensures that an oil film of the proper thickness can form at a given temperature and operating conditions, in order to keep the mating surfaces apart during hydrodynamic and EHL conditions. If the viscosity is too high or too low, excessive heat can build up, power consumption can become excessive and efficiency can decrease. The wrong viscosity can prevent the proper amount of oil from flowing to the contact zones, increase wear and reduce service life.
The OEM recommendations for lubricant viscosity are usually specified in centistokes (cSt) at 40 C (ISO Grading System), Saybolt Universal Seconds (SUS) at 100 F or by the AGMA Viscosity Grade. If no OEM recommendations are available, the correct viscosity may be determined using AGMA Guidelines found in Annex B of AGMA 9005-E02 and 9005-F16 standards. Another way is to refer to tables based on an industrial gear drive’s horsepower rating, reduction ratio, the speed of the gear drive and the type of lubrication method used. An alternative to using the tables is to calculate viscosity. One equation for determining kinematic viscosity at 40 C is:
v
40 = 7000/(V
1)
0.5
where V
1 = pitch line velocity of the lowest speed gear, feet per minute (fpm).
The AGMA Guidelines for selecting lubricant viscosity are based on pitch line velocity (PLV) of the lowest speed gear set rather than simple shaft speed. PLV indirectly characterizes contact time between gear teeth, and it takes into account the size and rotational speed of the gear. High PLV values are associated with light loads and high speeds, and, conversely, low PLV values are associated with high loads and low speeds.
Information on calculating PLV can be found in Refs. 1 and 2. Calculating PLV or use of equations may require the user to know the type of gear (
see Figure 4), number of teeth on the pinion and driven gears, gear ratio, pressure and gear helix angles, pinion speed (rpm), diametral pitch and operating and minimum expected ambient temperatures.
Figure 4. The type of gear determines what type of industrial gear oil is most appropriate. (Figure courtesy of Schaeffer Manufacturing Co.)
Operating conditions will determine what type of lubricant is selected. For example, inhibited type oils (R&O and/or AW) should be used for light and moderate loads, whereas anti-scuff (EP) type oils are used for moderate and high loads. Solid lubricants or soluble molybdenum compounds also can improve load-carrying capabilities. Anti-scuff oils should never be used in drives that use internal backstops, sprag clutches, ratchet mechanisms or load brakes; the EP additives in these oils can prevent these braking mechanisms from engaging properly, which defeats their purpose as safety features.
Whether you select an industrial gear oil by looking up the manufacturer’s recommendation, calculate it from first principles or go with something in between, customized to meet the requirements of your operation, knowing and understanding the properties of the formulation’s components can help you ensure that you get the best performance for your needs.
REFERENCES
1.
Johnson, M. (2008), “Gear drives: Selecting the correct lubricant,” TLT
64 (10), p. 24-28.
2.
Errichello, R. (1977), “Gear Lubricant Selection,” in Tribology Data Handbook, p. 781, edited by E. Richard Booser, CRC Press.