Commercial aviation lubrication
Debbie Sniderman, Contributing Editor | TLT Webinars July 2018
Few lubricants are asked to do so much—or endure so rigorous an approval process.
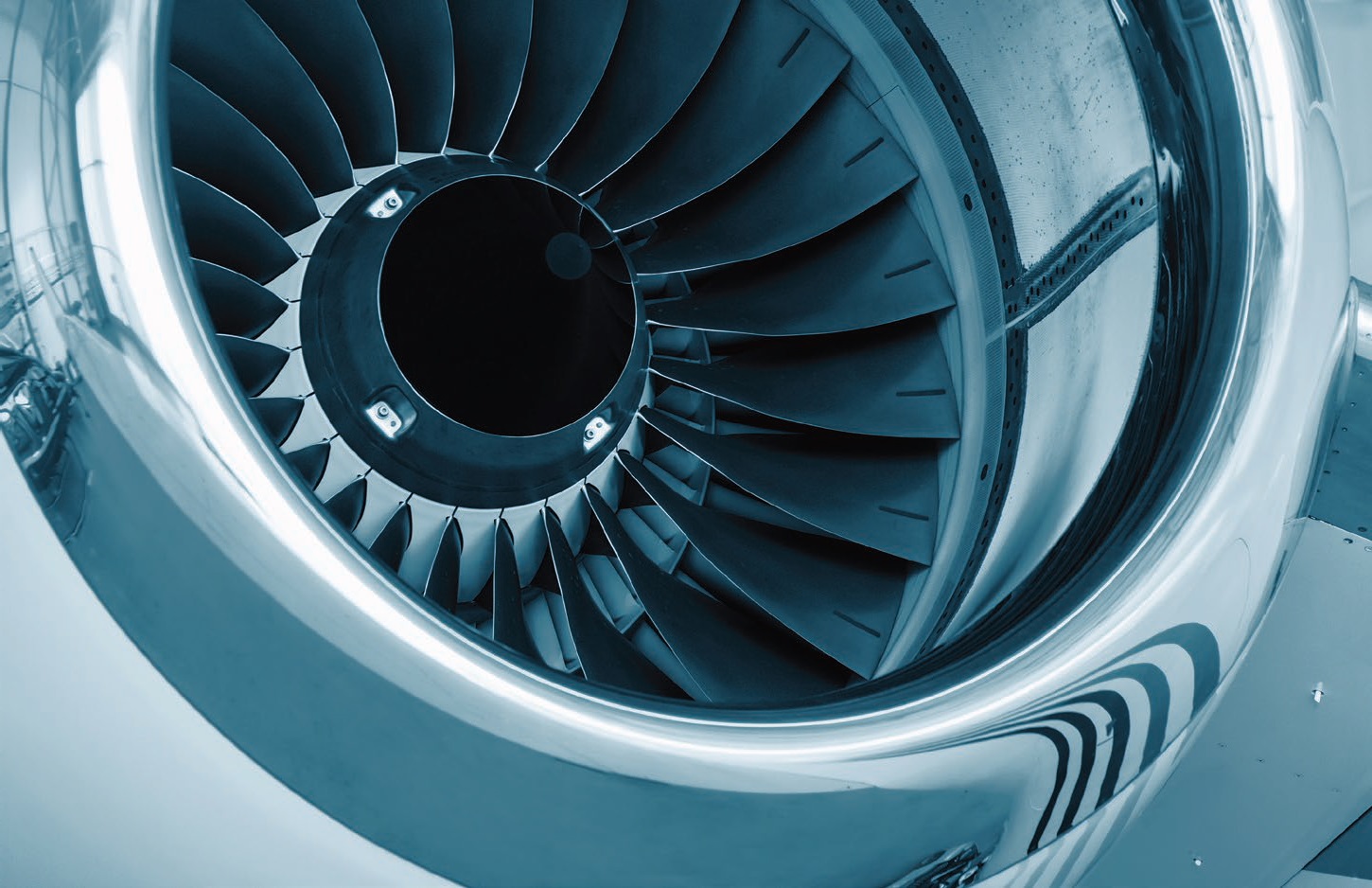
Photo courtesy of ExxonMobil.
KEY CONCEPTS
•
Aircraft lubricants experience performance demand far beyond those of other industries.
•
Hydraulic fluids used in modern commercial aircraft require fire resistance.
•
Airframe greases, turbine lubricants and hydraulic fluids must perform in extremely low temperatures while at altitude.
MEET THE PRESENTER
This article is based on a Webinar originally presented by STLE Education on Dec. 6, 2017.
Commercial Aviation Lubrication is available at
www.stle.org: $39 to STLE members, $59 for non-members.
Edward Barnes has 20 years of lubricants experience with ExxonMobil, almost all in aviation lubricant sales. He has served as an aviation lubricants global field engineer since 2011 providing technical support and training for ExxonMobil’s sales force, their aviation lubricant distributors and global customers.
Barnes is an active member of STLE and obtained his Certified Lubrication Specialist™ certification in 1999. He obtained his bachelor’s of science degree in marine engineering from California State University’s Maritime College. He worked as an engineering officer aboard commercial ships before joining ExxonMobil. He also has been an Federal Aviation Administration-licensed private pilot since 1985 and has had an interest in aviation since childhood. You can reach Barnes at
edward.l.barnes@exxonmobil.com.
Edward Barnes
Lubricants used in the aviation world are subject to significantly different performance demands compared to other industries. There are several themes making this industry unique. Lubricant technology development is a function of engine and airframe design changes. As aircraft equipment evolves, so do the lubricants. Turbine oils are exposed to much wider temperature ranges, from -73 C to 200 C. Also, the restrictive and regimented regulatory environment for aviation is like nothing else.
History of turbine engine lubricants
In the early days, natural mineral or castor oils lubricated the earliest piston engines. These lubricants have a long history that continued to develop through World War II. Mineral lubricants used in piston aircraft were acceptable for the requirements of the first turbine engines, so they were used in these early engines.
The jet age began in the late 1940s and set new demands on lubricants. The temperatures of gas going through the turbines increased. Along with higher turbine inlet temperatures, the lubricants themselves had higher temperatures. More capable chemistries were needed, changing the chemistries of lubricants.
By the 1970s, the Pratt & Whitney JT9D, one of the first high-bypass turbofan engines powering the Boeing 747, had turbine inlet temperatures at around 1,100 C. The technology continued to progress, and by the 1990s, for example, the GE90-115B, a Boeing 777 engine, had turbine inlet temperatures at around 1,500 C.
As engine temperatures increased, synthetic lubricant chemistries became necessary. Diester synthetics were first used in the 1950s, and their use continues to this day. Since the 1970s, however, polyolester synthetic lubricants have been more commonly used. The latest engines have temperatures above 1,800 C and some today are in the vicinity of 2,000 C. These engines use ceramic matrix composites because titanium can no longer be used for turbine components. The lubricant temperature is nowhere near the temperature of the gas. In the 1940s and 1950s, lubricant temperatures peaked around 100-120 C and have progressed up to about 200 C today (
see Figure 1).
Figure 1. Trends in turbine inlet temperature. (Figure courtesy of ExxonMobil/Dr. Yoshida, National Aerospace Laboratory Japan.)
Lubricants provide many important functions in jet engines. The most important is absorbing and removing heat from bearings. Heat is passed from the lubricant to the fuel, heating the fuel and cooling the lubricant. This fuel/oil heat exchange is important as the fuel tanks on aircraft are routinely exposed to cold temperatures at high altitudes.
Lubricants also are needed to reduce friction between moving metal parts and are expected to function across a wide temperature range. Turbine oils are expected to have a low temperature fluidity with pour point (< -54 C/-65 F) and low volatility at high temperatures since they will be exposed to temperatures at hot spots around 200 C. They should have high flash points (> 246 C/475 F) and function to carry particulate debris to oil filters for removal. Turbine lubrication also should protect engine parts from corrosion and resist and prevent deposit formation. They must also protect elastomeric seals, O-rings, gaskets and other seals in the engine, which can be particularly challenging based on the chemistry.
Jet engine lubricant chemistries: esters
Expected performance from today’s jet engine lubricants is well beyond the capability of petroleum-based oils. Today’s synthetic polyolester base stocks provide the needed exceptional thermal stability. Esters are not petroleum based; they’re synthetic fluids formed by reacting fatty acids and alcohols, producing water as a byproduct, which is driven off with heat.
One of the primary raw materials used as a source of fatty acids is palm kernel oil or coconut oil. Esters are polar molecules and are very hygroscopic, having a natural affinity for water and readily absorbing it from the atmosphere. This is why it’s important to keep water away from ester-based lubricants. Water contamination of ester fluids can cause hydrolysis, which may form acids, especially in sealed systems. This is a battle the aviation lubrication industry fights continuously.
To maintain product integrity, turbine lubricants are manufactured and used in metal cans. Lubricant containers should always be kept sealed until use. Opened containers should be used quickly and put into the application quickly to protect it from absorbing water from the atmosphere.
There are two basic types of esters in use today: diesters and polyolesters. Diesters are relatively simple molecules compared to the more complex polyester molecules. Diesters have slightly lower viscosities than polyolesters, and both have very good fluidity at cold temperatures compared to other lubricants. This is important because in aircraft they have to function routinely at temperatures of -40 C to -50 C.
Diesters are challenged in their resistance to oxidation, and their kinematic viscosity can change significantly when they oxidize. Diesters’ ability to resist oxidation is not as effective as polyolesters, which is why the trend toward using polyolesters as temperatures in engines and lubrication increase. Polyolesters also provide better resistance against deposit formation. Lastly, diesters have good compatibility with materials found in seals, base oil seals and additive packages in oils (
see Figure 2).
Figure 2. Commentary on the types of jet engine lubricants. (Figure courtesy of ExxonMobil.)
Specifications
Over the years lubricants have had to meet applicable specifications. The earliest turbine engines used mineral oil-based lubricants to meet the now obsolete mil spec MIL-PRF-6081. Although no manufacturers actively make products to meet the spec, it is still in effect for heritage and historical aircraft. Early jets flown in air shows will use 6081 spec fluids.
In the 1950s, as the technology of the turbine engine developed, “first generation” synthetic Type I lubricants were created under military guidance. Administered by the U.S. Air Force, the requirements became MIL-L (PRF)-7808. Most products are diester base stocks and some use polyolester base stocks. They offered improved thermal and oxidative stability and improved low-temperature viscosity over mineral oils.
7808 fluids continue to be used today in auxiliary power units (APUs), which are small turbine engines in the tail of most commercial airliners. While they don’t provide thrust, they provide electrical power for the aircraft on the ground and in emergency situations. Sometimes APUs need to start quickly at high altitudes in very cold temperatures, so having a low-viscosity turbine oil is important.
Second-generation Type 2 synthetic products arose in the 1960s to keep pace with engine technology developments that subjected lubricants to higher temperatures. The U.S. Navy wrote the MIL-L (PRF)-23699 specification as it searched for better performing lubricants. Type II lubricants are polyolester base stocks with significantly improved thermal stability. They also have higher viscosity of 5 cSt at 100 C and better antiwear protection and resistance to oxidation, meeting a higher set of demands than the 7808 fluids.
The 23699 specification continued to evolve, and in the early 1980s a new section was written, MIL-L (PRF)-23699 HTS, as the U.S. Navy desired better high-temperature thermal stability. Third-generation Type II-HTS lubricants offered enhanced performance over Type II oils for engines that worked at hotter temperatures. They used polyolester base stocks and had the same viscosity at 5 cSt at 100 C but had additive enhancements improving thermal and oxidative stability.
By the late 1990s, commercial manufacturers of engines and aircraft became dissatisfied with the MIL-PRF-23699 specification, and the fact that throughout most of the history of turbine lubricants the military made approval decisions. A civil specification emerged as OEMs wanted a say in the approval process and how lubricants would perform in their hardware (
see Figure 3).
Figure 3. Jet oil specification timeline. (Figure courtesy of ExxonMobil.)
A civil specification was necessary because military and civil gas turbine engine designs and requirements diverged, and both were operated and maintained very differently. For example, the F/A-18 Hornet, primarily used by the U.S. Navy but also used by the Marine Corps and others, has high performance in flight, a top speed of Mach 1.8 (1,381 mph) and two General Electric F404 engines that are typically not on the wing for long periods of time. They’re removed, cleaned, disassembled and inspected, and parts are replaced after on-wing engine operation < 2,000 hours.
While the maintenance approach for military engines is very intensive, the situation is very different for civil engines. For example, on a Boeing 737-800 with top speed Mach 0.82 (629 mph), less than military planes, it’s two CFM56-7B engines are left on wing for vastly longer periods of time without being removed. On-wing engine operation well exceeds 20,000 hours. Maintenance is handled on the airframe, but disassembly is only performed if necessary. Trying to keep the engines in civil service for long periods puts a different demand on the lubricant than in the military. Therefore, OEMs decided they needed their own specification.
The modern jet oil specification SAE AS5780 was written in the early 2000s to address next-generation engine requirements. The higher thermal stress in new engines demands lubricants with improved oxidative performance and load carrying without damaging seals and elastomers.
SAE AS5780 is essentially a supplement to MIL-PRF-23699, which is still in effect, and will be widely used into the future. SAE AS5780 has additional test requirements that reflect the reality of commercial aviation applications, including long-term seal compatibility and deposition tests. AS5780 creates two performance categories to qualify jet oils: standard performance capability class and high-performance capability class (
see Figure 4).
Figure 4. Military and civil gas turbine engine designs and requirements diverged, creating the need for new industry standards. (Figure courtesy of ExxonMobil.)
Commercial aviation lubricant-approval process
The aviation industry is highly regulated. Aviation turbine lubricant specifications contain requirements that are quite regimented compared to the other industries lubricant businesses serve. There are many requirements in the specifications. They contain compositional limitations, indicating exactly which chemistries can be used, and specifying that all additives must be ashless for aviation turbine oils. There is a long set of minimum physical and chemical properties to meet, which stipulate minimum performance requirements and thermal stability, tribological and deposition properties.
They also provide specific instructions on how to qualify a product and how to make changes to approved materials if a substitute material is needed. For example, if an additive supplier goes out of business, it specifies how to use the same additive from another supplier and the need to obtain permission from regulatory authorities to do so. If a significant formulation change is made, it specifies the need to fully re-qualify the fluid, requiring many years of testing.
Specifications also provide instructions on how to supply products to the U.S. government, requiring the authorized package types and labeling specifications. It provides instructions on how manufacturers are expected to certify the quality of their approved materials, vetting and investigating its own facilities and suppliers.
Qualifying a new product is now a decade-to-decades-long process. The starting point involves new product development, establishing product performance goals, deciding performance objectives to meet and performing research to decide base oils and a formulation. Once the formula is decided, qualification occurs with laboratory testing in house, bench testing in rigs and with regulatory agencies. Eventually qualification is performed against specifications, (e.g., MIL-PRF-23699F or SAE AS5780B).
Airframe and component manufacturers must then approve the oil for their hardware. Ground testing and/or on-wing service evaluations take place with the builders and manufacturers of engines, components and anything the jet oil will touch for what they require for approval. Their requirements vary. Some are happy with extensive ground testing in actual engines, but some require service evaluations on wings in actual aircraft. These could require 5,000 hours of experience in multiple engines, which could include the full removal and disassembly process, inspection of oil-wetted hardware as well as oil and filter analysis. Maintenance manuals also must be updated.
All data, documents and reports are submitted to regulatory authorities, either the Federal Aviation Administration or the European Aviation Safety Administration. They endorse the results and conclusions of the ground or on-wing testing, allowing the OEMs to issue final approval.
Final approvals often come in the form of a document known as a Service Bulletin. This is an official communication to commercial airlines that an oil is approved for an application. Getting through all the requirements for all of the engines and related hardware, done in parallel, takes 10-15 years. It is an enormous process to get a new oil approved in this industry.
Performance expectations
New turbine oils must be backward compatible with existing lubricants since the preference in the commercial aviation industry is for broad application. Managing multiple turbine oils for multiple engines becomes a significant challenge for airlines. So the ability to use a single lubricant in as much of the hardware as possible is greatly desired, making universal approval highly desirable.
General trends in engine temperatures in terms of gas path continue to increase, which may translate into still higher lubricant temperatures. While alternative chemistries are being assessed, the high heat capacity of esters is one reason why they continue to be used over alternate chemistries.
The materials in engines themselves also are changing. There are new alloys used in bearings, gears and accessory gear boxes, so new additive platforms are used. There’s also an increased use of non-metallic components. Oils must have improved compatibility with seals, paint and various composite materials. Additives are expected to produce less volatile antioxidants and better antiwear along with higher loading of degradation products while protecting elastomer seals over long periods.
The military sector is performing research on enhanced esters, ester-based oils that provide higher load carrying. There also are several exotic synthetic chemistries under consideration that have promise for turbine engine applications.
New oil technologies in commercial aviation follow the military experience where they have more latitude to take risks. The commercial aviation lubrication industry is extremely conservative and careful, which is why it can take 15 years for new products to get approved. It’s all about safety.
Aviation hydraulic fluids
Aviation hydraulic fluids have different sets of requirements. For mineral oil-based aviation hydraulic fluids, the military specification MIL-PRF-5606 is in effect. Products known as “red oil” that meet this specification are used in the landing gear of modern commercial aircraft as “strut fluids” and in older military aircraft.
Specifications for newer polyalphaolefin (PAO)-based fluids are given in MIL-PRF-83282 and are typical products found in most military aircraft. Another specification, MIL-PRF-87257, exists for lower viscosity fluids that are more flammable than 83282 fluids. Both are less flammable and more stable than MIL-PRF-5606.
Flammability is the most important reason totally different flame-resistant hydraulic fluids are used in modern commercial aircraft. Over the past 60 years as large commercial jets became more common, the phosphate ester chemistry evolved and is now what is used in all commercial large jets. Its use was prompted by higher aircraft speeds during landing with higher brake temperatures and more concerns about fires.
Hydraulic fluid used to actuate brakes and landing gear is pressurized from 3,000-5,000 psi. If it were to leak near an ignition source, like a hot brake, the resulting fog of fluid could ignite, hence the need for fire resistance.
Phosphate ester fluids use trialklyl phosphates that have excellent low-temperature performance and will function at temperatures as low as -60 C with pour points around -80 C. They offer many benefits over other types of hydraulic fluids. But the main reason they are used is for their fire-resistance properties. They are self-extinguishing fluids with high auto-ignition temperatures and zero flame propagation. Flames will not spread with these fluids (
see Figure 5).
Figure 5. Comparison of commercial aviation hydraulic fluids. (Figure courtesy of ExxonMobil.)
These products are qualified by commercial airline manufacturers since no military specification exists. They must meet airframe OEM testing against various specifications and specialized OEM tests such as hot manifold and high-pressure spray. Boeing BMS 3-11 is an example of an OEM specification.
Phosphate ester hydraulic fluids evolved similarly to turbine oils over time. Types I, II and III are no longer used. Type V fluids used today typically have longer fluid lives, and some have lower density and less weight, which is important in aviation. They also can manage the stresses of the more demanding 5,000 psi hydraulic systems on the Boeing 787, Airbus A380 and A350 aircraft (
see Figure 6).
Figure 6. Hydraulic fluid evolution. (Figure courtesy of ExxonMobil.)
Aviation greases
Grease is used in many airframe applications. It lubricates drive screws in hydraulically powered actuators that move flight controls and landing gear, track-ways for flaps, ailerons, leading-edge slats, rudder and elevator linkages.
Low-temperature performance is critical for aviation. At 30,000-40,000 feet in altitude, the air temperatures can go down -40 C to -70 C. Airframe grease has to function in application temperatures from -73 C to 121 C.
Airframe grease products must qualify against both military and OEM specifications to be sold. Military specs are MIL-PRF-23827 and MIL-PRF-81322, and an example of an OEM spec is the Boeing BMS 3-33.
Typically, PAO and ester-based oils with a lithium complex thickener are used in most aviation greases. There are some clay or synthetic clay thickeners used as well. They all have additives, and some specialized aviation greases use solid additives such as molybdenum disulfide.
Most aviation greases have consistency ratings between NGLI 1 and 2, with most around 1.5 due to low temperatures. Generally low-base-oil viscosities are needed for extremely low temperatures in airframe greases.
The commercial aviation lubrication industry is extremely conservative and very careful.
Photo courtesy of ExxonMobil.
Wheel bearing greases are used in sealed, tapered rolling element bearings on aircraft. There are two sets of bearings to handle axial loads in both directions. Since they’re sealed, there is no re-greasing while in service, but typically wheels are removed and overhauled on an accelerated schedule in commercial aircraft. Because of tire wear, the wheels are removed every 100-200 landings. While the tires are off being inspected, refinished, retreaded or reused, the bearings also are removed, cleaned and inspected. Those that are deemed airworthy are repacked with grease and put back into service. The grease is completely removed and replaced each time there is maintenance. This can be fairly often, every two to six months depending on the usage of the aircraft. Short haul carriers may make numerous landings per day, decreasing the service life.
Wheel bearing grease is exposed to conditions different from airframe grease. Wheel bearings experience higher temperatures, ranging from -54 C to 200 C, and are subject to higher loads compared to airframe applications. Base oil viscosity is higher in wheel bearing grease than airframe grease.
Wheel bearing grease products also are qualified by both military (MIL-PRF-81322) and wheel bearing and airframe OEM testing and specifications. A new specification (SAE AMS 3058) is currently in development.
For more information
ExxonMobil Aviation:
www.exxonmobil.com/aviation
ExxonMobil LinkedIn:
www.linkedin.com/company/exxonmobil-aviation
Debbie Sniderman is an engineer and CEO of VI Ventures, LLC, an engineering consulting company. You can reach her at info@vivllc.com.