The body mechanical
Dr. Nancy McGuire, Contributing Editor | TLT Feature Article June 2018
Inserting artificial joints into a biological environment can ignite the body’s ability to heal itself.
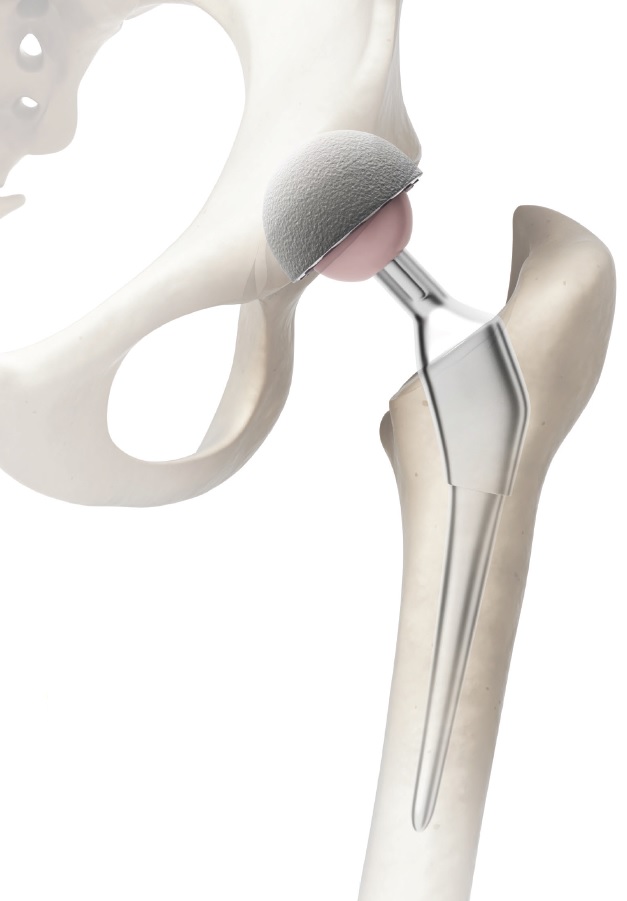
© Can Stock Photo / Eraxion
KEY CONCEPTS
•
More patients are getting joint replacements at younger ages and earlier disease stages.
•
Joint replacements often wear and corrode in the body very differently than in a lab environment.
•
Postponing or preventing the need to replace joints is an active area of research.
Back in the 1970s, the Bionic Woman’s medical implants gave her superhuman powers. People in the real world also had artificial implants, but these parts only restored some semblance of normal function to aging or injured bodies. Today medical implants still mainly repair and replace parts rather than enhance performance, but they come into play at a much earlier stage of damage, and their users typically enjoy a more active lifestyle than was previously possible.
Osteoarthritis, one major reason for joint replacements, is one of the 10 most common disabling diseases in developed countries. Worldwide, about 10% of men and 18% of women over age 60 show symptoms (
1), and more than a million joints are replaced with artificial implants every year (
2). Current estimates of the annual number of joint replacements in the U.S. put knees at 720,000, hips at 330,000 and temporomandibular joints (jaw hinges) at 3,000 (
3).
A new landscape
People have been getting hip implants on a mass scale since the late 1960s, says Jeremy Gilbert, Hansjörg Wyss Endowed Chair for Regenerative Medicine and professor of bioengineering at Clemson University in Clemson, S.C. Since then, surgeries have improved so drastically that they are now being used for a broader range of disease states, different stages of life and for very active people. Now people in their late 50s are getting hip implants, he says. Afterward they are still running and biking. Patient expectations are changing, he continues. People are less willing to just accept the pain—they want to fix the problem.
Gilbert notes that not only are implants being installed more often now, the kinds of operations now are more varied. Knee replacements are more common than hip replacements, he says, but people also are getting dental implants and replacements for various parts of the spine and shoulder. That’s not even counting nonstructural parts, like stents, which have their own tribological issues (
see Beyond Hips and Knees).
Beyond hips and knees
Here are some of the many areas where medical tribologists study friction and wear:
•
Knees
•
Hips
•
Shoulders
•
Temporomandibular joints
•
Vertebrae
•
Dental fillings, crowns and implants
•
Artificial limbs
•
Blood vessel prostheses
•
Heart valves
•
Pacemakers
•
Stents
•
Catheters
•
Heart assist devices
•
Pacemaker/defibrillator leads
•
Contact lenses
•
Hearing aids
•
Bone fracture fixation devices
•
Surgical instruments
•
Surgical gloves and gowns
•
Drug delivery patches
•
Bedsheets
•
Wound dressings and adhesive bandages
•
Diapers
•
Sanitary towels
Sources: Ref. 2, 3 and 10.
Markus Wimmer, the Grainger director of the Rush Arthritis and Orthopedics Institute at the Rush University Medical Center in Chicago, also has seen a trend toward joint replacement at earlier ages, but he says this isn’t an ideal approach. “Total joint replacement is a cure, but you lose a [natural] joint. Early intervention could postpone the time when you have to replace the joint,” he says.
However, better joint replacements are a positive development in a less-than-ideal world. When Wimmer began his career in the 1990s, joint replacements would typically last 10-15 years. That was often sufficient for the older, less physically active patients who would get the implants. When people started living longer, more active lives, “we wanted to extend longevity” of the artificial joints, Wimmer says. “From an engineering perspective, we did a pretty good job,” he adds, explaining that we now have better materials and a better understanding of wear mechanisms, which can extend a joint replacement’s service life well into a second decade.
Anatomy of a hip replacement
Hips, knees, shoulders, elbows, wrists—most of the joints in the body, in fact—are synovial joints. The ends of the adjacent bones are covered in tough, rubbery cartilage. Around the outside of the joint is a capsule made of strong, fibrous tissue, lined with a membrane that secretes viscous synovial fluid (
4).
Hip joints are a very sturdy type of ball-and-socket joint. The head of the femur bone forms a knob that fits into the hip socket on the lower part of the pelvis bone. The surrounding synovial capsule helps prevent an excessive range of motion that might dislocate the hip joint.
Artificial hip joint replacements have a ball attached to a metal stem using a modular junction that is designed to minimize vibration and other types of motion between the ball and stem (
see Figure 1). The top end of a patient’s femur is surgically removed, and the stem assembly is anchored within the hollow center of the femur using a pressure-type cross fitting. Earlier types of hip implants fixed the stem to the bone using cement, but this setup was prone to microscopic movements of the stem within the bone, which promoted fretting and osteolysis (bone resorption). An artificial cup lines the hip socket to protect the bone from wear.
Figure 1. X-ray image of a hip replacement joint. (© Nevit Dilmen.)
Various types of implants accommodate differences in weight and the intensity and type of physical activity among individual patients. The design is generally a tradeoff between biomechanical and tribological functions. Many athletes prefer hip implants with a larger femoral head ball that improves the joint’s stability and range of motion, but the larger contact area increases sliding distance and wear. Compare this with knee implants where better conformity between the articulating surfaces reduces contact stress but limits the range of motion and increases wear (
2).
Synovial fluid: Nature’s lubricant
Artificial implants use the same lubricant that natural joints do: synovial fluid. Synovial fluid separates the bones in a joint, reducing wear on the cartilage. It also absorbs shocks and filters out debris and inflammatory substances while letting nutrients pass through.
The main constituent of synovial fluid is sodium hyaluronate (also known as mucin). This long-chain organic polysaccharide compound forms tangled, web-like masses that absorb large quantities of water (
4). Hyaluronic acid or its sodium salt also is present in the vitreous body of the eye, the mucous membranes and the skin (
see Figure 2).
Figure 2. Hyaluronic acid. (Figure courtesy of Wikimedia Commons.)
Although research on the lubrication mechanisms of synovial fluid is ongoing, the consensus is that a combination of mechanisms interacts to provide lubrication (
5). Two systems within a synovial joint require lubrication: the soft tissue system and the interface between the cartilage caps on the bone ends. The hyaluronate component of synovial fluid sticks to the sliding surfaces of the soft tissues and keeps them separated, providing boundary lubrication. (Because joints oscillate and change their direction of motion, a hydrodynamic lubrication wedge never has a chance to form.) Lubrication at the cartilage interface relies on glycoproteins in the synovial fluid. At low loads, the glycoprotein provides boundary lubrication. At higher loads, the lubricating film is a combination of synovial fluid and interstitial fluid that weeps from the cartilage itself. Here, the hyaluronate may enhance the longevity of the lubricating protein and act as a spreading factor (
5).
Mathew T. Mathew, Cedric W. Blazer Endowed Professor in biomedical science at the University of Illinois College of Medicine at Rockford’s Regenerative Medicine and Disability Research Lab in Rockford, Ill., notes that lubrication conditions right after joint replacement surgery might be very different from those under ideal conditions. Shortly after surgery, the joint area is bathed in a “pseudo-synovial” fluid, he says, because the synovial capsule has been opened and the joint has been exposed. The surgical wound may contain blood and tissue debris, as well as the synovial fluid, and it may take a long time to regenerate the normal environment, he adds.
Implant materials
Any joint replacement must restore ease and range of joint motion, attach securely to the bone, be compatible with the surrounding biological elements and offer a long service life. Very low wear rates are typical for today’s artificial joints; only about 4% of joints need replacing (for any reason) after seven years (
1).
Different joints present different problems, each requiring their own material solution. Hips, knees and shoulders involve large bones and large ranges of motion. Ankles, elbows, wrists and fingers have smaller motions, and spinal discs and temporomandibular joints must be capable of complex motions in three dimensions.
Until about 2009, hip implants had both the ball and the cup made from stainless steel or a corrosion-resistant cobalt-chromium alloy. However, this type of interface tended to wear and release debris and ions into the joint, sometimes causing adverse reactions.
Today’s implants use various combinations of metal, ceramic and polymer parts and coatings that minimize wear and extend the life of the implant. The most common hip implant configuration today uses a metal ball with a cup made from (or lined with) ultra-high molecular weight polyethylene (UHMWPE). An alumina ceramic ball with a polyethylene cup, or both components made from ceramic, also are somewhat common (
1). There has been a recent resurgence of interest in metal-on-metal implants for younger, more active patients, but because these patients are more likely to have these implants for a longer period of time and put more wear and tear on them, the medical community is taking a cautious approach (
6).
Titanium alloys are good for spinal discs, but they require surface treatments to improve wear resistance. Joints where both contacting surfaces are made from zirconia-toughened alumina composite ceramics are becoming more popular, especially with younger patients, but these joints can squeak (
2).
Polyether-ether-ketone (PEEK) polymers are being investigated as longer-lasting replacements for UHMWPE. Carbon fiber-reinforced PEEK demonstrates good wear characteristics in simple geometry pin-on-plate studies and in total hip joint replacements. However, in low-conformity applications like knee joints, these polymers have wear rates that are significantly higher than for UHMWPE, and they have a tendency to delaminate and form surface cracks (
see Figure 3) (
7).
Figure 3. Knee replacement. (Figure courtesy of Wikimedia Commons. Author: BruceBlaus.)
Gilbert notes that despite recent developments in materials science as a whole, the selection of alloys used in today’s joint replacements remains small. Titanium, cobalt-chromium alloy, stainless steel and nickel-titanium alloys all work well inside the body. Cobalt-chromium, now commonly used for hip replacements, was developed in the 1920s for use in dentistry, he notes.
The FDA started regulating medical devices and biomaterials in 1976, and pre-existing devices and materials were grandfathered in. New devices that can demonstrate “substantial equivalence to a predicate device” go through a much shorter FDA approval process than do devices that are completely new, Gilbert notes. “We can innovate a little bit,” he says, but designs typically don’t stray too far from what was available in the 1970s because of the high investment and lengthy approval process needed to prepare a new device for market. New devices must be shown to be “safe and effective” (similar to the requirement for pharmaceutical products) rather than “substantially equivalent.”
New products that incorporate pharmaceuticals or stem cells into the device (combination products) must clear drug hurdles as well as device hurdles—a process that is “exponentially more difficult,” says Gilbert. The medical device field is shaped to a large degree by the regulatory process, he adds, but the process does catch harmful products before they get onto the market.
Patients can be reluctant to try new devices because of the risks associated with such an integral part of their daily lives. Issues with innovative devices can’t be tested easily, Wimmer says. Unlike new pharmaceutical products, which produce results almost immediately, joint replacement implants are meant to last for decades, and testing requires extended time periods, he adds, noting the need for better, more informative tests.
Unlike wear particles in a machine, micron-sized wear particles in a body not only cause friction, they can stimulate an immune response. Wimmer, who studies the macrophages that attack these foreign bodies, found that polyethylene particles produce a continuum of inflammatory responses.
Osteolysis is one such response; bone material breaks down and is reabsorbed by the body. The osteoclasts that normally digest proteins and minerals to assist in bone maintenance and repair can break down healthy bone tissue around a prosthesis, causing the device to loosen in its socket. The resulting abrasion causes both the bone and the implant to shed particles, causing even more inflammation.
Solutions to abrasion problems can work against solutions to fatigue problems caused by loading and vice versa. More highly cross-linked forms of polyethylene can reduce adhesive wear but are more brittle, Wimmer says. Cobalt-chromium is especially hard on polyethylene, requiring a highly cross-linked polymer or a vitamin E radical scavenger. It’s a matter of finding the optimal tradeoff between the competing factors, he says.
Corrosion mechanisms
The metal alloys used for joint implants are extremely corrosion-resistant in a laboratory environment. Inside the body, it can be a different story.
Fretting corrosion, which occurs as the result of minute vibrations in the contact area between two materials under load, is only recently receiving intensive study, partly as a result of the problems encountered with metal-on-metal hip joint replacements (
2). Fretting occurs in the absence of lubrication, and it involves contact between surface asperities and movements on a scale of nanometers to millimeters. Oxidized debris particles are often harder than the bulk surface, and these particles can abrade the surface, accelerating the fretting process (
8).
The interface between the ball-and-stem tapers of a hip replacement joint can fret easily, says Wimmer. Cobalt-chromium resists abrasion and corrosion well under normal conditions, but the alloy degrades when both processes act in synergy. Even small quantities of debris and ions can cause inflammation, which is one of the reasons that metal-on-metal joints are under scrutiny, he says. The body responds to excessive amounts of metal degradation products by forming areas of inflammation and masses of necrotic tissue that look like tumors around the metal debris, he adds.
Switching to ceramics, which are more inert to corrosion, is one solution. There are better, more forgiving materials out there, says Wimmer, but the industry is reluctant to innovate because of regulatory issues.
Since metal-on-metal hip implants have fallen out of favor and been replaced with other materials, sliding corrosion of the head on the cup has been minimized, but micro-motions at the head-neck and neck-stem connections still sometimes result in fretting corrosion. Mathew's student Dmitry Royhman found that variations in loading, micromotion amplitude and pH influence fretting corrosion at modular junctions. Another of his students, Maria Runa, studied fretting at the stem-bone junction and found more osteolysis than corrosion.
Tribocorrosion in a biological environment has recently emerged as another area warranting further attention. Tribocorrosion is a synergistic phenomenon—tribology accelerates corrosion and vice versa. “It’s more than just an additive effect,” Mathew says. For example, abrasion erodes the oxide surface from a titanium implant, exposing a fresh metal surface that oxidizes. The electrochemical repassivation process doesn’t always balance out the mechanical depassivation process, so repassivation can sometimes produce more erosion, releasing materials into the surrounding media.
Mathew’s research group made a mechanical tribocorrosion hip simulator in 2009, and they found that rubbing increases corrosion by an order of magnitude (
see Figure 4). Stress corrosion is a known phenomenon, but contributions from tribological processes within electromagnetic fields are still not clearly understood, he says.
Figure 4. Hip implant simulator. (Figure courtesy of Mathew T. Mathew.)
Implant manufacturers and researchers perform tribotests to estimate the service life of their products, Mathew says, but it is difficult to simulate the complex biological joint environment. Sometimes implants that performed well in laboratory tests last only a matter of months inside the body. Inside the body, implants must contend with acute or chronic infections and the resulting immune response, various medications, chemicals from tobacco smoking, loading and stress from obesity and a range of other individualized factors, he says.
Gilbert’s group is investigating the role of inflammation on promoting corrosion. The body is a salt water environment, he says. Implants release particles and ions, which the body recognizes as foreign material. The response mechanisms haven’t been fully established, he says, but toxicity is one possibility. A typical immune response releases chemicals that might promote the release of more particles and ions from the implant, creating a feedback loop, he says.
Reactive oxygen species stand out as an area of interest, Gilbert says. Immune cells generate hydroxyl radicals, hydrogen peroxide, hypochlorous acid, peroxynitrites and superoxide anions. “There’s a whole mix of these oxidizing agents that go after foreign bodies,” he says. In the lab, when you put reactive oxygen species into salt water, it has a dramatic effect on alloy corrosion.
“The hypothesis that inflammatory species can alter the corrosion process is still pretty new,” he says, noting that his group is still in the early stages of modeling the processes. They search the literature to find which cells make specific reactive oxygen species and in what concentrations, and they make similar mixtures in the lab for use in corrosion testing and testing for passive oxide film formation. So far they have tested hydrogen peroxide and hypochlorous acid, and they found that both species enhanced corrosion.
Gilbert’s group also found that electrochemical reduction reactions produce hydroxide ions and reactive oxygen species that can promote corrosion. Bone cell cultures on titanium or cobalt-chromium substrates placed into a reducing environment can die off within a matter of hours. These reduction reactions haven’t been looked at much, Gilbert says. “How this happens inside the patient isn’t really understood,” he adds, but tribocorrosion may play a role. His group is investigating how electrical potentials arising from tribocorrosion of metal-on-metal contacts can kill cells, even outside the rubbing area.
During a study on fretting corrosion, Gilbert’s group used a potentiostat to control the electrical potential in a cell culture (
see Figure 5). The cells lived until the potential crossed a threshold, showing that the electrical potential rather than the wear debris was killing them. “We’re not seeing this in patients yet,” he says, adding that the conventional view focuses on wear particles and ions. These things do cause harm, but they aren’t the whole explanation, he says.
Figure 5. MC3T3-E1 cell culture. (Figure courtesy of Jeremy Gilbert. Image taken by Shiril Sivan, Ph.D.)
Bodies are more self-correcting than machines, Gilbert continues. The alloys we use for joint replacements also are used in saltwater environments like the oil platforms in the North Sea, where fretting is usually a minor annoyance. However, oil platforms don’t have immune systems or redox homeostasis mechanisms like the body does. The body tries to maintain a balance, Gilbert says. There are mechanisms for control and feedback, and when you get outside the range the body can handle, cells start to die.
Wear measurements
Unlike an engine or a gearbox, you can’t just shut down a human body to do maintenance or drain out all the synovial fluid and replace it with a test lubricant. Medical tribologists face distinctive limitations on observing part failures in progress and examining mechanical systems
in situ. Many humans would object to being observed all day by a video camera or an engineer, or to carrying out their daily functions while feeding data to a laboratory instrument. By the time patients come to the hospital for an implant replacement, they are often in distress and in no mood to be interviewed and examined by anyone other than their physicians.
Thus, medical engineers typically resort to using mechanical wear simulators during the process of getting joint replacements approved for clinical use. Various tribometric instruments have been adapted for use with joint implants and tissue cultures, with promising results (
see Wear Testing Methods). Accelerated testing hasn’t proved feasible, however, because it doesn’t accurately represent clinical use. Computer simulations could help eventually, but the biomechanical environment around the joints hasn’t been fully characterized, and the degree and type coupling between interactions at the contacting surfaces and interactions at the musculo-skeletal level isn’t well known (
2).
Wear testing methods
Wear testing takes a variety of forms designed to assess surface topographies and wear progression for the complex shapes of teeth, knees and hip joints. Here are several methods currently in use.
Source: Ref. 11.
Laboratory tests must try to replicate conditions in the body as closely as possible, but it’s not a simple task. Take lubricants, for example. Artificial implants use the body’s own synovial fluid as a lubricant. A typical healthy knee joint will contain 1-2 mL synovial fluid, says Wimmer. Wear tests in the lab require 150-200 mL or more, so it’s hard to collect enough synovial fluid for lab testing. Also, it’s very expensive and it decomposes. The hyaluronic acid in synovial fluid gives it a high molecular weight and drives the viscosity properties, but also it’s very expensive.
Wimmer’s research group uses a surrogate material based on bovine serum, which consists mostly of albumin protein. Bovine serum is plentiful, economical, thoroughly studied and has ISO standard preparation methods. The protein content of Wimmer’s test fluid is similar to that of synovial fluid, but the rheology is different.
Wimmer’s group tested worn implant components, tissue samples and wear particles retrieved from live patients who were getting replacement implants, and they compared these to tissue samples and implant components that hadn’t failed, retrieved post-mortem from other patients. Even though the rheology for bovine serum was different than for hyaluronic acid, the wear mechanisms were similar. He cautions, however, that, “you have to be careful about extrapolating results” from one implant material to another. Results of bovine serum tests with polyethylene don’t necessarily carry over to ceramics, metals or other combinations of materials, he says.
Mathew’s research group uses a tribocorrosion apparatus in an incubator to simulate
in vivo conditions for tissue cultures. You can put a new material into a machine and it works well, Mathew says, but individual patient anatomies may produce different results. It’s difficult to set up models that replicate conditions within the body, so interactions among clinicians, biotechnologists and bioengineers are key to understanding the system.
Mathew’s group gets patient histories, symptoms and demographics along with the retrieved implants they study. They don’t typically interact directly with the patients, however, because of privacy concerns and the need for physicians to act quickly in an emergency situation.
Wimmer notes that the medical community feels that it has solved the problem of “total joint wear” (premature failure), and this failure is usually because of infection rather than tribology. Joints also fail because they weren’t installed correctly or the surrounding bone reorganizes, causing the prosthesis to become loose. Tribology research was an active area for the past 20 years, but the field has matured, he says, and the focus has shifted to lowering infection rates, which have stayed constant at about 1%-2% for many years.
Most of the tribological issues occur in knees, ankles and hips, says Wimmer. There aren’t as many tribological issues with shoulder joints, although some new research is looking into this area as well. Shoulder joints consist of several small bones rather than two large bones, and they have much more soft tissue, so finding a way to anchor a device to the bone is a more complicated problem. Also the range of motion is much greater for shoulders than it is for hips and knees (
see Figure 6).
Figure 6. Shoulder replacement. (Figure courtesy of Wikimedia Commons. Author: Lucien Monfils.)
On the horizon
Prosthetic devices relieve pain and restore function (at least partially), but they are artificial devices inserted into a biological environment. Much of today’s research focuses on integrating medical devices and biomaterials into a living system and harnessing the body’s own capabilities to help it restore itself.
Very few tissues in the body naturally regenerate, Gilbert says—wounds form scar tissues rather than regenerating healthy tissue. He cites research on regenerative methods—still in the early stages—that attempt to direct stem cells to regenerate bone cells. To do this successfully, he says, you need to develop substrates that act as scaffolding for the growing cells and drugs or other cues that prompt the cells to grow in a certain direction and control immune and inflammatory responses.
Tissue engineering, using the body’s own tissues to regrow natural parts, is in its infancy. Regenerated cartilage can be used to repair injuries, but it’s not used much now for osteoarthritic applications, says Wimmer.
Genetic engineering also is in its early stages. Wimmer cites one research group that uses knockout mice, which have one specific protein-making gene activated or deactivated. This group is looking at lubricin, a protein that is important in boundary lubrication at the cartilage surface. The proteoglycan 4 gene (PrG4), which encodes the instructions for making lubricin, could be an important target for treatment, Wimmer says.
Helping people take care of their own joints so they don’t need artificial replacements is another active area of research. A self-monitoring hip implant that could signal your cell phone and alert your doctor could catch problems in the early stages, Gilbert says. His group is currently working on ways to monitor implants for corrosion. Sensor development and intensive data analysis could provide solutions in the near term, while tissue engineering and regeneration technologies are coming up to speed, he says, but if devices are to provide useful feedback, you have to find out what signals are important.
Wimmer and his colleagues are currently working on shoes with pressure-detecting insoles that provide feedback to a patient’s smartphone. The aim is to teach people to walk differently in order to mitigate the effects of arthritis (
9).
Medical imaging techniques could provide physicians with early warnings of impending joint failure. Noninvasive,
in situ diagnostics like functional MRI scans or Raman spectroscopy could provide valuable information on chemical processes as they happen, but we’re not there yet, says Wimmer. However, this type of investigation could bring the tribosystem into the lab, allowing researchers to watch healthy patients and those with arthritis as they perform various activities.
Just as oil analysis can warn of impending machine problems, developing tests for biomarkers could give an early warning for inflammation, infection and immune responses, says Mathew. His research group is working on a biosensor for metal ions and particles in the blood. “It’s like a glucometer but for metal,” he says. Particles from human joint implants have a lot of unknowns, he says. They aren’t just metal particles, and they can range in size from 10-200 nm. Metal particles and ions can form organometallic complexes with proteins, and nanoparticles can incorporate into cells, causing them to die.
Taking a pharmaceutical approach could be useful. Wimmer cites the example of today’s eye drops, which supplement or replace the lubricating fluids on the surface of the eye. He notes that people currently get hyaluronic acid injections for their knees to reduce joint friction, but in reality the pain may decrease because hyaluronic acid scavenges radicals produced by inflammation.
Room for improvement
Reducing implant infection rates is one area that is ripe for improvement, says Gilbert, adding that this rate has held steady at 1%-2% for the past 25-30 years. Gilbert is investigating ways to use electrochemical potentials, which have proven so damaging to human cells, to kill bacterial biofilms on metal implant surfaces. “It’s a lemons-to-lemonade approach,” he says.
Preventing the need for implants altogether remains a promising but elusive goal. Most of the time by the time the patient comes in, the damage is already done. Wimmer asks, “How can we identify disease markers that could serve as an early warning?” We don’t have one single marker for osteoarthritis at present; there’s no clear signal for why certain people develop this and some don’t, he adds. “It’s very complicated in the body,” he says. Biology, chemistry, mechanics—“this all needs to come together.”
Gilbert notes that researchers spend their time studying what can go wrong with implants and trying to prevent or mitigate those problems. But overall only a small percentage of joint implants develops problems. “Implants provide tremendous benefits to millions of people,” he says. Still, problems must be addressed. Patients have high expectations, Gilbert says. “They need to understand these things are amazing but imperfect, and they still present a technical challenge.” Sometimes things happen even when you do everything right, he adds.
“You have to remember that you’re working for the people,” and keep a focus on the patient, Mathew says. The patient wants to know, “What’s going to happen to me?”
REFERENCES
1.
Ian Hutchings FREng (March 2016), “Fifty years of tribology,” first published in Ingenia, the Royal Academy of Engineering magazine, Issue 66. Available
here.
2.
Z.M., Jin, Zheng, J., Li, W. and Zhou, Z.R. (2016), “Tribology of Medical Devices,”
Biosurface and Biotribology,
2, pp. 173-192. Available
here.
3.
American Joint Replacement Registry report via Markus Wimmer. Available
here.
4.
TRB Chemedica UK Ostenil, Patient Information (product website). Available
here.
5.
Lipowitz, A.J., (1985), “Synovial Fluid,” Chapter 86, in Textbook of Small Animal Orthopaedics, eds. Charles D. Newton and David M. Nunamaker, J. B. Lippincott Co. Available
here.
6.
Howard, J.J. (2016), “Balancing innovation and medical device regulation: The case of modern metal-on-metal hip replacements,”
Medical Devices,
9, pp. 267-275. Doi: 10.2147/MDER.S113067. Available
here.
7.
Brockett, C.L., Carbone, S., Fisher, J. and Jennings, L.M. (2017), “PEEK and CFR-PEEK as alternative bearing materials to UHMWPE in a fixed bearing total knee replacement: An experimental wear study,"
Wear, Vol. 374-375, pp. 86-91. Doi: 10.1016/j.wear.2016.12.010. Available
here.
8.
ASM Handbook on Fatigue and Fracture, click
here.
9.
Rush University Medical Center news release (Sept. 26, 2017), “Walk on the Mild Side.” Available
here.
10.
Gresham, R.M. (2017), “Medical Textiles and Tribology,” TLT,
73 (7) pp. 22-23. Available
here.
11.
Valigi, M.C., Logozzo, S. and Affatato, S. (2017), “New Challenges in Tribology: Wear Assessment Using 3D Optical Scanners,”
Materials,
10 (5), p. 548. Doi: 10.3390/ma10050548. Available
here.
Nancy McGuire is a free-lance writer based in Silver Spring, Md. You can contact her at nmcguire@wordchemist.com.