Automotive industry lightens up
Dr. Nancy McGuire, Contributing Editor | TLT Feature Article November 2017
The trend toward lightweight vehicle parts is driving changes in manufacturing processes and metalworking fluids.
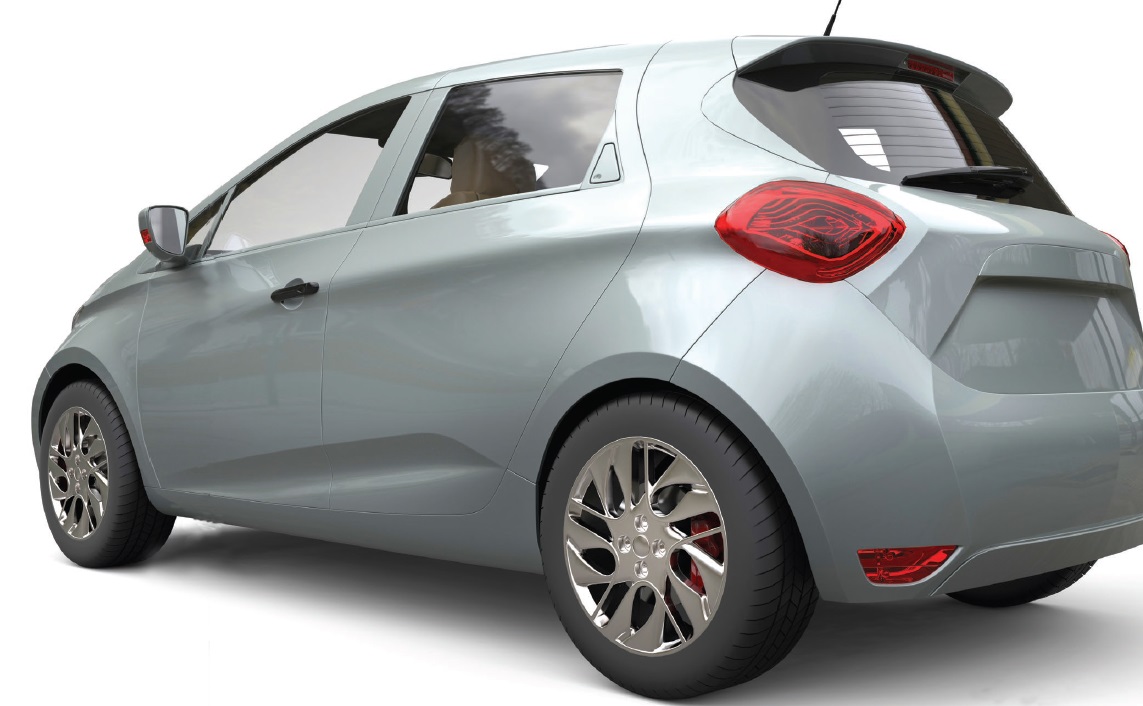
© Can Stock Photo / Trimitrius
KEY CONCEPTS
•
Lighter metals and alloys pose new challenges in corrosion prevention and high-temperature processing.
•
Metalworking fluids are being reformulated for high-speed processes, downstream compatibility and regulatory compliance.
•
Global competition, industry consolidation and customer demand ensure that these trends are likely to continue.
THE AUTOMOTIVE INDUSTRY IS UNDER PRESSURE FROM ALL DIRECTIONS. Global competition drives faster, more efficient manufacturing operations while keeping costs low. Government regulations set standards for the kinds of chemicals that can be used in manufacturing processes and for the fuel efficiency and safety of new vehicles on the market. Customers demand reliability and high performance; company mergers and acquisitions take technological advances from smaller product lines into the mainstream; and the aerospace industry constantly introduces new processes and materials.
Much of the drive over the past decade has been toward reducing vehicle weight. The use of thinner and stronger materials results in lighter vehicles, which use less fuel; however, the move toward lighter materials comes at a cost. The materials themselves can be more expensive, as in the case of advanced alloys, but manufacturing processes also must be adapted to work at higher temperatures or to work with metals and alloys that oxidize more easily than steel, for example. Companies adopt various strategies that often combine new and old technologies, processes and materials. “One size does not fit all,” says David Budai, automotive industry manager, Americas, for Houghton International.
Some OEMs focus on cost; for instance, if they are making vehicles for a mass market and need to keep the price down, they might be slower to adopt materials that require a change in processes or the purchase of new equipment. Others go for the lightest weight with space-age approaches for their pricier vehicles. Budai spends much of his time helping OEMs with vehicles that are still in development and might not hit the marketplace until 2022 or 2023.
Over the past several years, Ted McClure has seen pronounced trends toward global competition, which puts pressure on domestic operations to increase their productivity. McClure, technical resources manager for Sea-Land Chemical Co., deals mainly with clients who work with metal-forming operations in the automotive industry. Companies must produce parts faster and more reliably with less down time, he says. At the same time, they are working with new materials, new tools and tool coatings, new environmental regulations and latest performance requirements like Corporate Average Fuel Economy (CAFE) standards.
Domestic OEMs use different strategies for lightweighting to meet CAFE standards, says Budai. Some are making lighter engines, and some are making lighter structural parts like body panels to get the CAFE numbers up. For example, Ford’s best-selling vehicle, the F-150 pickup truck, uses aluminum body panels, and the manufacturer will be transitioning this to their other vehicles during the next four or five years, Budai says. According to Ford’s Website, the F-150’s cab and box are made from “high-strength military grade aluminum alloys.” That, and the high-strength steel in the frame, reduce the pickup’s weight by 700 pounds compared to the previous generation of trucks. Other manufacturers are heading in that direction as well but maybe not as quickly as Ford, Budai says.
OEMs are looking for strong materials with the desired degree of ductility and good wear resistance. “We’re seeing more aluminum alloys containing titanium now in specific engine components like power train valves, camshafts, pins, crankshafts and parts like exhaust and intake valves,” Budai says. His colleague Yixing (Philip) Zhao, senior research scientist and innovation team leader, research and technology, at Houghton, adds that newer aluminum alloys have higher strength, different compositions and harder surfaces. The aerospace industry is the biggest user of titanium right now, Zhao says, but the knowledge that fluid developers gain in developing lubricants for this market eventually transfers to other industries.
STEEL STILL AROUND
The auto industry is changing quickly; newer vehicles are made using thinner, stronger steel panels. Moving to high-strength steels is “a huge change in the way cars are made,” McClure says. “It’s really phenomenal,” he adds. In 2005 mild steel made up about 85% of the mass of a typical auto body, with the rest being high-strength steel. As of 2015, typical auto bodies were about 40% mild steel and more than half high-strength steel. Lighter metals like aluminum and magnesium make up the remainder, as much as 10%, but these metals are gaining, he says.
Mild steel has a low carbon content—a few tenths of a percent to a few hundredths of a percent. Mild steel is ductile, machinable and easily welded, but it has a low tensile strength, typically less than 200 MPa. Thinner sheets of steel can make a lighter vehicle, McClure says, but it takes a stronger steel to achieve the same or better crash worthiness. These high-strength steels have tensile strengths in the range of 210-550 MPa. In this range, the steel is ductile enough to be shaped but strong enough to meet performance standards. Some advanced third-generation steels have tensile strengths exceeding 1,000 MPa, but they still have “decent formability,” McClure says (
see Figure 1).
Figure 1. The “banana diagram” is a common way to present the tradeoff between a metal or alloy’s ductility (as percent elongation) and strength. This diagram compares magnesium alloys and 5xxx, 6xxx and 7xxx series aluminum alloys with various types of steel. (Figure courtesy of R. Schneider, B. Heine and R.J. Grant (2014), Mechanical Behaviour of Commercial Aluminium Wrought Alloys at Low Temperatures, Light Metal Alloys Applications, Dr. Waldemar A. Monteiro (Ed.), InTech, DOI: 10.5772/58362. Available at www.intechopen.com/books/light-metal-alloys-applications/mechanical-behaviour-of-commercial-aluminium-wrought-alloys-at-low-temperatures.)
Generally steel loses ductility as it gains strength, McClure says, making it harder to form. In addition, the less ductile steel tends to spring back after it is pressed, making dimensional accuracy difficult. Solving this problem is an active area of research, he adds. High-strength steels are more expensive and harder to work with, which drives up production costs. Higher tool temperatures from working with these steels and the higher stresses involved in forming parts requires tools, tool coatings and lubricants that can stand up to these conditions, he says.
ALUMINUM RAMPING UP
High-strength aluminum presents some of the same ductility and spring-back problems as high-strength steels, along with higher costs, McClure says. These factors had been holding back the use of aluminum, but now that manufacturers are moving toward higher-strength materials there is less of a barrier to using aluminum (
see Aluminum 101).
ALUMINUM 101
•
Aluminum is abundant; it makes up slightly more than 8% by weight of the Earth’s crust.
•
Aluminum has good electrical and thermal conductivity.
•
Low modulus of elasticity: aluminum parts can yield elastically rather than being cut cleanly.
•
High coefficient of linear expansion: cutting processes that generate a lot of heat can cause workpieces to expand.
•
When aluminum is exposed to air, it spontaneously forms an oxide layer that is impervious to further oxygen penetration.
•
Aluminum is relatively expensive to extract from the ore.
•
Aluminum can be recycled easily; nearly 75% of all aluminum ever produced is still in use.
•
Cast aluminum alloys commonly use recycled aluminum, but with the proper attention to scrap alloy chemistry and cleanliness, high-end wrought alloy components also can incorporate recycled aluminum.
•
In 1980 containers and packaging (largely beverage cans) was the largest U.S. market sector for aluminum, followed by construction.
•
By 1995 transportation was the largest sector of the U.S. market for aluminum (27%), followed by containers and packaging (24%).
•
In 2015 the North American transportation industry used 9.2 billion pounds of aluminum—35.8% of total North American shipments.
•
Anodizing aluminum forms a thicker oxide layer, which can be dyed various colors.
•
Aluminum alloys containing appreciable amounts of silicon are difficult to anodize.
•
Cast aluminum alloys often contain 5%-12% silicon to increase wear resistance, but the silicon can abrade tool surfaces.
Source: Ted McClure, Sea-Land Chemical Co.
See references 1-7.
“Aluminum is expanding into more applications,” at least for some vehicles, says Dianne Carmody, Americas marketing director, global product management adjacent businesses, for Houghton International (
see Figure 2).
Figure 2. Many vehicle manufacturers are incorporating more high-strength steel and aluminum into their vehicle frames. Gray and purple are conventional and ultra-high-strength steel, respectively. Blue, green and red are aluminum sections, sheets and cast parts, respectively. Black is carbon fiber reinforced plastic and yellow is magnesium. (Figure courtesy of Houghton International.)
Randy Sebastian, Houghton’s technical program manager, research and technology, concurs. Some semi-truck manufacturers have reduced the weight of their diesel engines by about 50% by going from cast iron to compacted graphite iron (CGI) for their engine blocks, but this material is very difficult to machine, he says. Engine makers are now moving toward aluminum for their gasoline engines. Mitsubishi and Cummins have already begun introducing aluminum diesel engines, and other aluminum engines are in the prototype stage now, he adds.
FLUIDS EVOLVE
As materials and alloys change, the processes for working with them must change as well. Fluid formulators are looking for fluids with a long service life to reduce downtime and increase productivity. Their customers are going toward faster speeds, using smaller sumps. Everyone wants low-foaming fluids that don’t stain, emulsions that remain stable in hard water and additives that don’t promote microbial growth (while still complying with regulations on allowable biocides). Not only that, but manufacturers are looking for compatibility with everything the fluid comes into contact with. “You have to look way downstream,” McClure says.
“All the products have a place in the industry,” says Zhao. North American and Asian companies use more water-based fluids, but European companies also are starting to move away from straight oils and toward water-based fluids.
Water-based fluids are complex packages, though, and you have to balance all the components, Zhao says. Operations using high-strength materials generate more heat, and water-based fluids are better coolants than straight oils. Newer fluid technologies build in better detergency (prevention of particle agglomeration and coating formation on surfaces), dispersion and wetting, all of which are needed for the lighter metals.
Some operations still require straight oil metalworking fluids (MWFs), says Mark Soder, Houghton’s director of technical service, research and technology. Smaller parts and those requiring tight tolerances or a smooth surface finish are examples of this. Magnesium work often uses neat oil because it requires a lubricant that won’t stain or release hydrogen gas. However, even here the trend is toward water-soluble fluids for insurance reasons. Emulsion-based fluids are less flammable than neat oils and you don’t have to evacuate the mist like you do with oils, he says.
HIGH TEMPERATURES, HIGH PRESSURES
High-speed machining fluids for working with softer metals must have good cooling and lubricating properties to prevent excessive heat formation due to friction. Softer metals can expand and lose strength if the temperature rises too high. However, many of the new high-strength alloys must be heated to make them ductile enough to work with.
Aluminum alloys that were previously only used in the aerospace industry are beginning to be used in the automotive industry, McClure says (
see Figure 3). The 6,000 and 7,000-series alloys are normally formed at elevated temperatures, 260-400 C (500-750 F). This limits the kind of lubricants you can use for forming operations, and you don’t always even use a lubricant. When a lubricant is used at these elevated temperatures, he says, it may include more solids, inorganics and phosphates.
Figure 3. Aluminum alloys that formerly were used only in aerospace applications are finding their way into automobile manufacturing.
© Can Stock Photo / Leaf
Lubricants for the automotive industry have to perform at higher temperatures and pressures, McClure says, while maintaining compatibility with adhesives, cleaners, primers and welding. Straight oils don’t encounter the biological or hard-water problems that water-based fluids do, he adds, but they often become volatile at higher temperatures, producing fumes and smoke, and they may be prone to oxidation.
Oxidized lubricants are harder to clean off a part, McClure adds. Lubricants and other fluids must not interfere with adhesives, including structural adhesives, which are seeing increasing use as a replacement for welding. This is an important research area, McClure says (
see Adhesives).
ADHESIVES
High-strength steels and aluminum parts are more difficult to weld than mild steel. Epoxy structural adhesives are sometimes used in addition to or instead of welding in situations where welding is difficult.
Epoxies are good sound deadeners and sealers, and they increase structural rigidity.
2015 automobiles typically had about 55 linear feet of structural adhesives. 2017 models have about 68 linear feet, and this is predicted to go up to 87 linear feet by 2020.
Some high-end, aluminum-intensive vehicles contain as much as 600 linear feet of structural adhesives.
Source: Ted McClure, Sea-Land Chemical Co.
Dave Slinkman, senior vice president, global research and technology, for Houghton, notes that rolling high-strength steel or aluminum sheet requires greater force, but at the same time body panels need a smoother surface finish than other parts might require. “We’ve had to make significant changes to the lubricity of our formulas to be able to work in those environments,” he says.
Zhao elaborates: Working with lighter, stronger metals increases the need for boundary/EP lubrication additives. The hard surfaces of high-strength steels, aluminum alloys and the titanium alloys used in the aerospace industry require a different lubrication mechanism, he says. “We test additives by themselves and as a part of formulation packages for specific applications, and we use design of experiments to optimize our formulations,” he adds.
TAMING ROGUE IONS
Hard-water cations can cause emulsions to separate, and they can leave mineral deposits on tools and workpieces. Calcium and magnesium are the usual suspects, but aluminum ions can cause hard water as well. Cast aluminum alloys contain magnesium, notes McClure, and magnesium is particularly hard on water-soluble cutting fluids. Formulations must contain additives that can stabilize emulsions in this environment.
Lightweight metals like aluminum and magnesium are more easily corroded than steel is, and their protective oxide coatings are stable over a much narrower pH range than are the iron oxides. Semi-stable emulsions that deposit an oil coating on the workpiece are one way to prevent corrosion, but the oil film must then be cleaned off before coatings or paints are applied.
Even a small amount of corrosion can present a problem in the form of staining. Aluminum, magnesium and their alloys are prone to staining. Aluminum is a more reactive metal than steel, so processing fluids must contain corrosion inhibitors to prevent staining, says Hoon Kim, senior principal R&D scientist with Chemetall (
see Corrosion Inhibitors: A Primer).
CORROSION INHIBITORS: A PRIMER
Various classes of chemical compounds can prevent corrosion and staining on aluminum metal and alloys. Each has advantages and disadvantages.
Inorganic compounds
•
React with ions in solution before they can reach the aluminum surface
•
Many of these compounds (including arsenates and hydrazine) are toxic
Heterocyclic organic compounds
•
Good for copper-containing aluminum alloys
•
Prevent galvanic corrosion caused by close contact between aluminum and copper
Sulfonates and phosphates
•
Work well as corrosion inhibitors
•
Encourage microbial growth: biocides required
•
Solutions with less than 2% phosphates prevent stains effectively
•
Sulfonate-containing fluids can have an unpleasant odor, and they can corrode copper
Amine carboxylates
•
Very effective at keeping aluminum from staining
•
Don’t require biocides (if the proper amine chemistry is selected)
•
pH buffering action, which improves the overall stability of the MWF
•
Relatively large amounts required, typically as much as 10%-15% of the formulation
•
Changing to a different amine has a big impact on the buffering and stain prevention properties of the fluid
Complex esters
•
Small oxygen content requires a relatively large amount (6%-7%) in the fluid to be effective at preventing stains
•
High cost means that they are used mostly as lubricants rather than corrosion inhibitors
Silicates
•
Similar chemically to phosphates and sulfates
•
Microbes don’t use them as food
•
Inexpensive
•
High oxygen content means you can use less to get the same level of corrosion protection
•
Tend to form gels in water-based fluids
•
0.8% tetraethyl orthosilicate (TEOS) can prevent staining on aluminum alloys without forming a gel
Silanes
•
Lower oxygen content than silicates
•
Less prone to forming gels but must use more to prevent corrosion
•
Bonding at least one long-chain organic group to the silicon atom further disrupts gel formation (but can drive up the cost of the additive)
Compounds with branched organic substituents
•
Degree of branching has little effect on pH
•
Highly branched organic substituents are significantly better at preventing aluminum staining than their straight-chain counterparts
Amine-functionalized organosilicates
•
Still under development as fluid additives
•
Combine the beneficial properties of amines with those of silanes
•
Do not require biocide
•
Good stain protection.
Source: Hoon Kim, Chemetall.
Ferrous metals corrode in neutral to acidic environments, but not in highly alkaline environments, because their surface oxide layers are stable at high pH. Aluminum readily forms a protective oxide layer, but the oxides are only stable in a fairly narrow pH region on either side of neutral. This can present a problem when MWFs are kept at a pH above 9 to protect expensive steel tools, Kim says. At this high pH, aluminum workpieces stain, and highly alkaline fluids can dissolve the protective aluminum oxide layer as fast as it can form, so MWFs require corrosion inhibitors.
Processes that generate fresh metal surfaces by machining or grinding away the oxide layer require the use of fluids that protect these surfaces from direct contact with tool surfaces or chips to prevent welding or adhesion, often referred to as “sticking.” Because stainless steels, aluminum and titanium form a pure oxide skin, the sticking effect is more pronounced for these metals than for steel or copper (
8).
Mild corrosion leaves a yellow or gold stain on aluminum. This can occur when you’re using the right type of MWF, but it’s been in use for too long and the corrosion inhibitor is becoming depleted. Using MWFs meant for ferrous metals can cause more severe corrosion that leaves a gray or black stain. Even the right MWF can stain an aluminum workpiece if certain additives (triazine biocides, for example) raise the pH of the fluid too high (
3).
SEMI-SOLIDS? SOLIDS? NO LUBRICANT?
Not every metal-forming operation uses a lubricant, McClure says. High-strength steels containing boron (in the gigapascal tensile strength range) are formed at 650-850 C (1,202-1,562 F), and they are quenched in the die to form very strong parts. Conventional lubricants can’t withstand these temperatures, he adds.
Magnesium is often machined dry, without a cutting fluid, because it doesn’t need the cooling or the lubricating effects. MWFs are sometimes used in the more difficult machining operations (e.g., deep hole drilling) or work at high spindle speeds, but here the MWF is mainly a coolant, especially to keep the chips from igniting (
see Magnesium 101) (
4).
MAGNESIUM 101
•
Magnesium is the third most commonly used structural metal after steel and aluminum.
•
Magnesium is mainly used for making aluminum alloys.
•
Magnesium is the lightest metal used in the production of structural components.
•
Magnesium has extremely high strength-to-weight ratio.
•
Magnesium is used for making wheels, radiator supports and engine blocks.
•
Magnesium alloys are among the easiest metals to machine.
•
Magnesium offers high thermal conductivity and dissipates heat rapidly.
•
Magnesium is sometimes machined dry without using any sort of metalworking fluid.
•
Water-based fluids can stain magnesium more easily than they can aluminum or zinc.
•
Direct contact between magnesium and water can release flammable hydrogen gas. Aerating the fluid can minimize this.
•
Newer water-based fluids use slightly unstable emulsions that deposit a thin oil coating onto the metal surface, which limits the contact with water.
•
Magnesium corrosion generates heat, so drums full of scrap or chips can catch fire.
•
Magnesium fires are very hot and difficult to extinguish—using water or a carbon dioxide fire extinguisher actually feeds the flame, sometimes explosively so.
Source: Randy Sebastian, Houghton International.
See references 4 and 9.
Some aluminum-forming operations use semi-solid, “half-hard” lubricants, McClure says. Semi-solid or solid lubricants overcome some problems because they stay put rather than migrating within a coil, preserving an even coating, and they can provide excellent lubricity. However, these coatings can be costlier to apply and, like the traditional fluids, they must be compatible with assembly, cleaning and painting.
ADAPTABILITY ISSUES
Some fluid formulations are advertised as multipurpose, suitable for a wide range of metals and applications. Does one size really fit all? “It’s always a balancing act,” McClure says. You can optimize your formulation for one type of metal or one operation, but “users are reluctant to inventory too many different fluids in the plant.” Thus, it’s a trade-off between simplifying inventory and optimizing performance. “If it’s a critical operation, you might use a fluid that’s specific to one type of material in a particular type of operation,” McClure says.
A new lubricant has to be approved for use before it is introduced onto the factory floor in an OEM plant, where making changes involves a long process, McClure says. A lot of research is being done, but changes in the factory tend to be incremental to prevent issues in the manufacturing process. For example, fluids must be compatible not only with workpieces (which can have various surface treatments, including the galvanized coatings on high-strength steel) but also perform well with the various tool materials and die coatings that they come into contact with. “It’s constantly evolving,” McClure says (
see Figure 4).
Figure 4. Houghton chemist Scott Lay inspects an advanced high-strength steel cup made with the state-of-the-art Valley Forge Labs 40-ton stamping press that the company uses to evaluate new technologies. (Figure courtesy of Houghton International.)
ENVIRONMENTAL CONSIDERATIONS
Much of the drive toward lighter metals is driven by the push toward greater vehicle fuel economy. Carmody says that in the U.S., CAFE standards are one factor in the drive toward lighter weight. Another is a trend toward consolidation in the transportation sector. As companies like Ford and Audi undergo mergers and acquisitions, they share their technologies with the vehicle lines in their new corporate environment.
Environmental considerations affect fluid formulations as well. Carmody says there’s a trend toward “minimum quantity lubrication” (MQL) and away from “flood lubrication.” This approach typically requires a change in manufacturing equipment as well as different fluid formulations, she says, so operations generally don’t adopt this approach until they are ready to replace their equipment.
Dry machining has proven successful in numerous operations, but MWFs are still required for applications that require cooling and lubrication, including titanium milling for aerospace applications or working with compacted graphite iron for engine parts. Using an MWF reduces tool wear (thus reducing tool replacement and disposal costs), as well as producing better parts by reducing residual stresses, dimensional errors and poor surface finish. Fluids also allow processes to be run at faster speeds without building up excessive heat. These factors can more than balance out the environmental impact of using fluids in the process (
10).
Environmental considerations also affect what additives you can use. For example, McClure says, conventional EP additives, including sulfurized, chlorinated and phosphorus-bearing additives, can react with steel but not necessarily with nonferrous metals, zinc-coated steels and tool coatings. OEM plants have gotten away from chlorine, he adds, but it’s difficult to replace chlorinated fluids in some severe operations, including fine blanking. It can be particularly difficult to replace chlorine for severe operations on some stainless steels as well, he says.
Regulatory requirements differ from one region to another, says Slinkman. Older fluids, including those that contain chlorinated paraffins, are acceptable in one region, but fluid suppliers in other regions have to make significant changes to their products. Products for sale in Europe must comply with the Registration, Evaluation, Authorisation and Restriction of Chemicals (REACH) regulations. “We’re having to conduct a complete review of our product lines to make sure that we are compliant,” Slinkman says. “We expect this to have a huge impact on the industry, in terms of what products are available for these new uses.”
Often an old fluid that works well in one region of the world will have to be heavily reformulated for sale in another region, Slinkman continues. “That’s a big change from what we’ve seen.” To keep up with the regulatory changes while reducing the amount of reformulation work, they start their product development by finding “a basket of raw materials that we can use in five or six different regions,” he says.
Carmody notes that many of Houghton’s customers want products that they can use in most or all of their locations around the world. Sometimes, however, specialized regional formulations are required to accommodate differences in things like water quality, she adds.
MWF MARKET
Competition and regulation come together to drive changes in MWFs, McClure says. Changes in fluid formulations are driven not only by new demands from parts manufacturers (who, in turn, are driven by marketplace and regulatory demands)—they also are pushed by new product offerings from the chemical companies’ R&D labs. “I hear from both sides,” says McClure.
It’s not enough just to watch overall industry trends, says Chuck Faulkner, Houghton’s product marketing manager for metal forming, forging and heat treatment. “We watch it all,” he says. Individual OEMs do things differently. “We work with colleagues all around the world, and they all work with different materials and processes,” he says.
In a 2016 press release, Gaia Franzolin, global marketing manager for Swedish specialty-oil manufacturer Nynas, cited a predicted 5.5% global increase in demand for private cars and light commercial vehicles over the next five years. She predicted a concurrent growth in demand for MWFs, driven in part by new materials like the lightweight aluminum and titanium alloys that are being adopted for weight reduction and fuel efficiency. “Ultimately it’s important to remember that technological advancements also bring the need for improved MWF performance (longevity and stability). And their lower consumption will be compensated by higher costs and improved tool life,” she says (
11).
LOOKING TOWARD THE FUTURE
Budai notes that the trend to reduce weight in automotive powertrains continues, with the downsizing of engines from V8 to V6 and I4 engines and the addition of turbocharging. The smaller turbocharged gasoline engines generate more heat, which raises the under-hood temperature. A few years ago, he says, OEM manufacturers were replacing steel engine parts with thermoplastics, but now some of the commonly used thermoplastics can’t always deal with the higher heat generated by these smaller, hotter running engines economically. So aluminum and magnesium are being considered in some cases as more cost-efficient alternatives to replace the higher-priced high performance thermoplastics in engine parts.
Kim sees a role for polymer composites; he notes that the aerospace industry uses carbon fiber reinforced plastic (CFRP) composites, in addition to aluminum, titanium and high-strength steel. Automaker BMW uses some CFRP composites in its passenger compartments but not for structural components, Kim says. Because CFRPs are so easy to form, the aerospace industry has moved from steel to aluminum and then toward CFRP. It’s possible that the automotive industry could follow this trajectory as well.
As challenging as today’s high-speed, high-temperature manufacturing processes are, even bigger changes are on the horizon. Carmody notes that the aerospace industry is beginning to use 3D printing for prototyping, and the medical industry already uses 3D printing with metals and composites to make joint replacements. Printed automotive components would require no processing fluids, and complex parts could be formed as one solid piece, with little to no need for machining, forming, stamping or other processes that generate heat and waste material.
The challenges involved in integrating these new materials and processes into efficient, reliable vehicles are becoming increasingly complex. Fluid formulators who work with the automotive industry clearly have their work cut out for them.
REFERENCES
1.
The Aluminum Association. (November 2016), “Industry statistics, facts at a glance – 2015. Available
here.
2.
Aluminum Recycling. Available
here.
3.
Byers, J. P., ed., (2006),
Metalworking Fluids, Second Edition, CRC Press: Boca Raton, FL. ISBN-13:978-1-5744-4689-0.
4.
Evans, R. and eds. Astakhov, V. P. and Joksch, S. (2012), “Metalworking fluids (MWFs) for cutting and grinding: Fundamentals and recent advances,” Woodhead Publishing Series in Metals and Surface Engineering Series #48,
Elsevier Science, pp. 29-42. ISBN-13: 978-0-8570-9061-4.
5.
Martinsen, K. and Gulbrandsen-Dahl, S. (2015), “Use of post-consumer scrap in aluminum wrought alloy structural components for the transportation sector, 22nd CIRP Conference on Life Cycle Engineering,”
Procedia CIRP,
29, pp. 686-691. Available
here.
6.
Plunkert, P. A., (2006), “Aluminum recycling in the United States in 2000,” U.S. Geological Survey Circular 1196-W. Available
here.
7.
U.S. Dept. of Energy, (July 1997), “Energy and Environmental Profile of the U.S. Aluminum Industry.” Available
here.
8.
Schulz, J., Brinksmeier, E. and Meyer, D. (2013), “On the interactions of additives in metalworking fluids with metal surfaces,”
Lubricants,
1, pp. 75-94. DOI: 10.3390/lubricants1040075. Available
here.
9.
Byers, J. (2010), “Corrosion issues and test methods,” Presentation at the STLE Annual Meeting and Exhibition.
10.
Skerlos, S. J., Hayes, K. F., Clarens, A. F. and Zhao, F. (2008), “Current advances in sustainable metalworking fluids research,”
International Journal of Sustainable Manufacturing,
1(1-2), pp. 180-202. Available
here and
here.
11.
Nynas AB. (Oct. 31, 2016), “Growth opportunities in the MWF market,
News from Nynas (press release). Available
here.
Nancy McGuire is a free-lance writer based in Silver Spring, Md. You can contact her at nmcguire@wordchemist.com.