Nanocars: Moving toward use in ambient conditions
Dr. Neil Canter, Contributing Editor | TLT Tech Beat October 2016
Researchers are figuring out how to design nanocars that can overcome sticky spots and other barriers.
KEY CONCEPTS
• The focus of ongoing research to develop nanocars now has the objective of determining how they can operate under ambient conditions.
• Confocal single molecule fluorescence microscopy can track nanocars as they move across a clean glass surface or a polyethylene glycol modified glass surface.
• The reason that most of the nanocars stopped moving after 24 hours is due to the accumulation of hydrocarbon-like contaminants on the glass surface.
ONE OF THE LONG-TERM OBJECTIVES IN DEVELOPING microelectromechanical systems (MEMS) is to transport specific molecules to particular locations. This approach led to the development of nanocars more than 10 years ago.
A TLT article published in 2006 details some of the initial work done in the preparation of a motorized nanocar (1). The nanocar is a complex molecule that is 3-by-4 nanometers in size and has two key components essential in facilitating motion. Alkynyl groups are used to prepare the vehicle’s axles because they enable the axles to freely spin at room temperature. The other component is what functionality to use in preparing the nanocar’s wheels.
James Tour, T.T. and W.F. Chao professor of chemistry, professor of material science and nanoengineering and of computer science at Rice University in Houston, says, “We initially started working with fullerene wheels. Our belief was that the spherical orientation of the 60 carbon atom fullerene molecule should facilitate smooth rotation of the four wheels used.”
However, problems were encountered when a motorized version of the nanocar was synthesized. A Feringa motor was utilized because it can be excited by light at a wavelength of 365 nanometers. Unfortunately, exposing the motorized nanocar to light did not lead to motion because the energy transferred quickly to the fullerene rings preventing the nanocar from moving.
Initial work was done on a gold surface in ultrahigh vacuum using scanning tunnel microscopy (STM) to monitor the nanocar’s progress. Tour says, “This setup is not a realistic way to enable nanocars to operate under ambient conditions. We decided to move to a hydrophilic glass surface or a glass surface further modified with polyethylene glycol, which is known to be non-sticky.”
The next material used in preparing nanocar wheels was para-carborane. Improved movement on glass was realized with 25% of the nanocars. Tour says, “But para-carborane wheels are too hydrophilic leading to hydrogen bond interactions between the boron-hydrogen bonds of the spherical para-carboranes and the hydrophilic surface that either reduces motion or prevents the nanocar from moving at all.”
Eventually, the researchers moved to using adamantane wheels. Adamantane is more hydrophobic leading to less interaction with the hydrophilic, glass surface and better movement. Tour says, “Approximately 50% of the nanocars equipped with adamantane wheels move because they do not stick on the glass surface.”
All of this initial work has been done in a controlled, atmosphere environment where the nanocars are operated under ideal conditions. To be useful, nanocars need to be adapted for use in ambient conditions.
Tour says, “The challenge we face with operating nanocars in ambient conditions is the presence of more and more barriers that can prevent them from moving. Nanocars will face constant bombardment from different types of molecules constantly dropping on them. Many of these molecules are the size of trees, relative to a car, making it very difficult for the nanocars to negotiate their way through this potential obstacle course.”
A second challenge involves how to monitor the movement of the nanocars. Tour says, “Monitoring of nanomaterials is harder than synthesizing them.”
STM is an excellent way for monitoring nanomaterials in a vacuum but is ineffective in the presence of air. This technique also emits energy influencing the movement of the nanocars. A new way to track nanocars is needed and has now been developed.
SMFM
Tour, Gufeng Wang, assistant professor of analytical chemistry in the department of chemistry at North Carolina State University in Raleigh, N.C., and their colleagues have determined that confocal single molecule fluorescence microscopy (SMFM) can be used to track nanocars moving across a clean glass surface or a polyethylene glycol-modified glass surface under ambient conditions. Tour says, “We placed a fluorescent marker on each nanocar and used signals collected by the microscope to locate each nanocar.”
In this study, the nanocars are allowed to undergo “direct diffusion” across the hydrophilic glass surface. The cars are not equipped with a motor.
Initially, an estimated 50% of the nanocars are able to move across a clean glass surface at an initial diffusion coefficient of 7.6 +/1.5 x 10-16 square meters per second. After 24 hours, the researchers determined that approximately 5% of the nanocars are still moving but the diffusion rate has dropped to 1.8+/-0.4 x 10-16 square meters per second.
The track of a nanocar is shown in Figure 2.
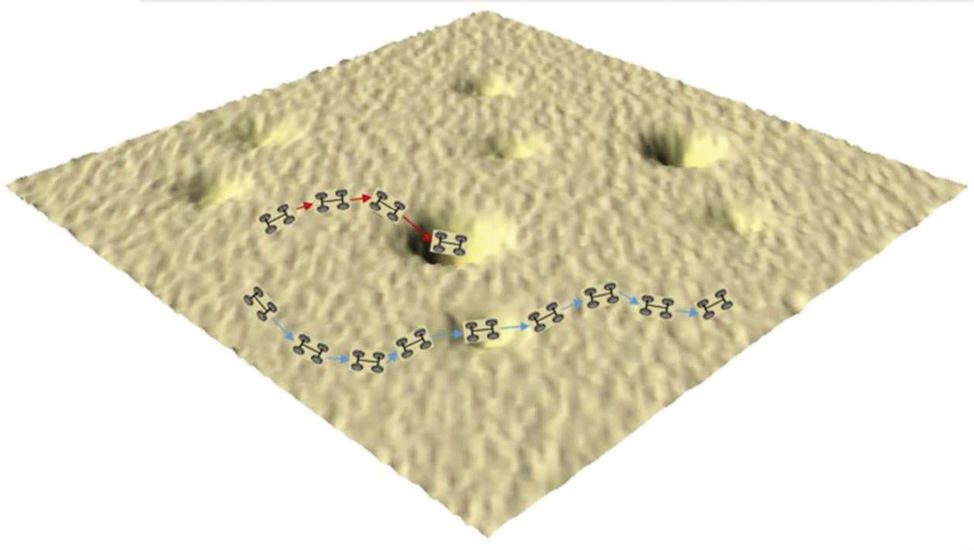
Figure 2. The track of a nanocar across a hydrophilic glass surface under ambient conditions is shown. (Figure courtesy of Rice University.)
The key factor slowing down the nanocars over time is the accumulation of molecules on the glass surface. Tour says, “An increase in the hydrophobic nature of the surface due to the adsorption of hydrocarbon-like contaminants from the air and also possibly the condensation of silanol groups on the glass produces a surface that is more compatible with the hydrophobic adamantane wheels leading to interactions slowing down the nanocars.”
The researchers hypothesize that the slowing down of the nanocars is due to a sticky-spots model characterized by the increase in the number of locations on the surface where the adsorbed hydrocarbon contaminants form sticky spots that either slow down the nanocars or stop them permanently. A uniform friction model also is proposed that claims that a uniform force on the surface is the direct cause of slowing down the cars. This model is not favored because the researchers observe that nanocar movement is reduced in localized areas on the glass surface.
Tour says, “Over the past years that we have been building and studying nanocars, we are making progress in finding more effective ways to monitor their movement and are getting closer to our goal of having them move efficiently on surfaces in ambient conditions.”
The next step of the work is to study motorized nanocars moving across the hydrophilic glass surface. Tour says, “We are close to this goal as we are now working on installing light-powered motors that can move at three million rotations per second, giving them a good chance to overcome the hydrophobic obstacles present on the glass surface.”
Additional information on these new nanocar developments can be found in a recent article (2) or by contacting Tour at tour@rice.edu.
REFERENCES
1. Canter, N. (2006), “Developing a motorized nanocar,” TLT, 62 (8), pp. 10-12.
2. Chen, F., Lopez. V., Jin, T., Neupane, B., Chu, P., Tour. J. and Wang, G. (2016), “Moving kinetics of nanocars with hydrophobic wheels on solid surfaces at ambient conditions,” Journal of Physical Chemistry C, 120 (20), pp. 10887-10894.
Neil Canter heads his own consulting company, Chemical Solutions, in Willow Grove, Pa. Ideas for Tech Beat can be submitted to him at neilcanter@comcast.net.