Friction-reducing feathers
Evan Zabawski | TLT From the Editor December 2012
Swimming can be such a drag. Unless you’re a penguin.
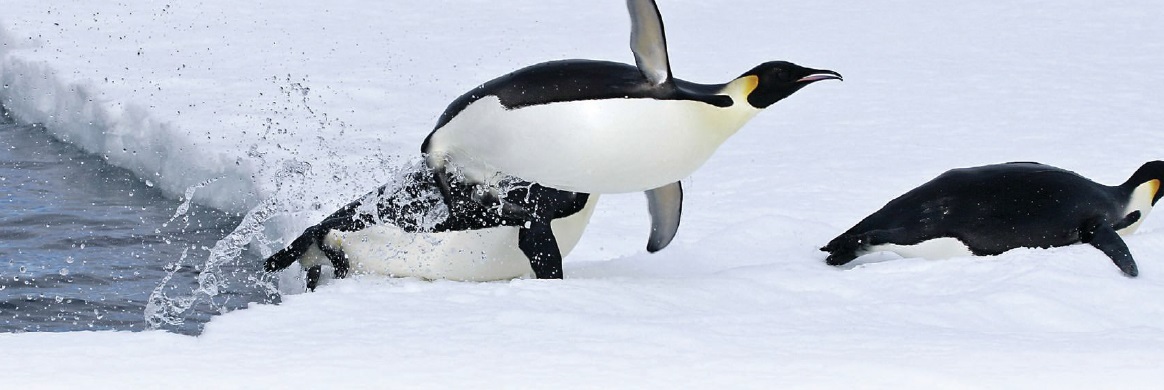
www.canstockphoto.com
WE ALL KNOW THAT PENGUINS CAN’T FLY. They can’t walk very well either, but they’re pretty good swimmers. Perhaps their best talent, which is a relatively unknown skill, is their ability to lubricate themselves in order to swim faster.
A typical Emperor penguin can swim at speeds between three and six miles per hour (a medal-winning Olympian swims just over four miles per hour in short distances). When penguins leap from the water onto an ice flow, they manage to get their speed up to more than 12 miles per hour before breaking the surface. This burst of speed is sometimes necessary to evade a predatory seal or to overcome several feet of ice, and only recently have we come to understand how this is possible.
Competitive swimmers know that the greatest force working against them is friction in the form of drag, since water is approximately 800 times denser (and 55 times more viscous) than air. Various attempts have been made to reduce drag while swimming. The first and foremost being body physics. When swimmers dive into the water, they assume a torpedo-like shape: one hand on top of the other on outstretched arms, elbows squeezed tight against the head, straight-body and legs terminating with pointed toes.
Optimal stroke technique comes next. A swimmer must overcome the body’s impractical design of rounded arms and legs to produce the best stroke possible. The last aspect to consider is the body’s outer layer. Most swimmers will shave their bodies to remove their speed-robbing hair, and some may don a full-body swimsuit. In contrast, a penguin’s body is naturally shaped for optimal speed already, is equipped with efficient flippers and yet is covered in feathers. Feathers seem like an unlikely advantage, but recent studies have shown it to be true.
Scientists Roger Hughes, John Davenport, Marc Shorten and Poul Larsen have discovered the secret of the Emperor penguin feathers. Just before a penguin makes its leap, it surfaces and preens its feathers. Their feathers are very downy near the base and this preening action traps air. Then the penguin will dive to a depth of 40-50 feet, which allows the air trapped in their feathers to compress.
Another feature of their feathers is that they terminate in a flexible membrane attached to muscles, which allow the penguin to alter the position of its feathers. So once the penguin begins its ascent, it flexes these muscles to pull the feathers closer to its body, which pushes the air through the feathers where the downy mesh creates <20 µm pores that act like a diffuser. The escaping air forms a thin layer of microbubbles all over its body that greatly reduces the drag on the penguin without grossly affecting buoyancy, allowing it to accelerate more easily. This low-friction coating is a temporary form of self-lubrication that gives a flightless bird the ability to fly out of the water to safety.
Though it’s a recent discovery, we have been aware of the concept for some time. One of the earliest examples can be found in a patent application by Sir John Isaac Thornycroft in 1877 for air-lubricated ship hulls. His design was never applied to a practical application, but developments over the next 75 years inspired the design for hovercrafts.
During the Cold War, Russians were able to increase the speed of their torpedoes from 30-40 miles per hour to over 200 miles per hour by having a cavitator create a supercavity of bubbles for the rocket-propelled torpedo to travel through.
Some recent developments have been made regarding air lubrication for container ships and supertankers, and perhaps one day soon we will see a significant reduction in the energy requirements for ocean-borne transportation.
Evan Zabawski, CLS, is a senior reliability specialist in Edmonton, Alberta, Canada. You can reach him at evan.zabawski@gmail.com.