Special Additive Report: Metal Deactivators: Inhibitors of metal interactions with lubricants
Dr. Neil Canter, Contributing Editor | TLT Tech Beat September 2012
These additives provide superior performance in preventing dissolved meals from negatively impacting lubricants.
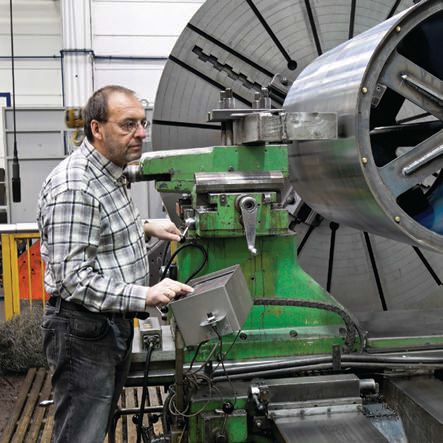
KEY CONCEPTS
•
The increasing use of nonferrous metals in lubricant systems means that metal deactivators are growing in importance to minimize such negative effects as staining and corrosion.
•
Metal deactivators form inactive barrier films on metal surfaces to counteract the catalytic effects of metals on oxidation and corrosion.
•
Selection of metal deactivators is based on a number of factors including the metal to be protected, the basestock used, frequency of the application, length of time it needs to be effective and any additional properties needed for the application.
THE GROWING USE OF NONFERROUS METALS, INCLUDING THOSE BASED ON COPPER AND ALUMINUM ALLOYS, is placing more emphasis on the need for additives to minimize such negative effects as staining and corrosion. A second issue is that these metals are also catalytically active and can trigger reactions that can facilitate the decomposition of the lubricant.
Metal deactivators are available to the lubricant formulator to help with these two issues that are gaining in importance. One other aspect that metal deactivators assist with is minimization of galvanic corrosion or bimetallic corrosion that can take place between different metal alloys.
A number of additive types are used as metal deactivators. The traditional components are nitrogen-based additives such as aromatic triazoles, substituted thiadiazoles and azoles.
Metal deactivators are highlighted in this month’s TLT additive issue to provide further details about their function, how they should be selected for a specific application, evaluation testing that can be done to measure their performance and future trends affecting their use.
To seek a broad range of opinions, TLT interviewed the following representatives from seven metal deactivator suppliers:
•
Dr. Ian Macpherson, senior marketing manager, & David Lindsay, technical manager-metalworking fluid additives, Afton Chemical Corp.
•
Pat Brutto, applications R&D leader, Dow Personal Home & Industrial Care.
•
Derek Phillips, global commercial manager-metal protection, & Seth Crawley, technology manager, The Lubrizol Corp.
•
Bridget Crocker, technical manager, PCC-Chemax, Inc.
•
Dr. Ron Pearson, R&D director, PMC Specialties Group, Inc.
•
Thomas Rossrucker, VP technology, and Dr. Sandra Horstmann, product specialist and application engineer-lubricant division, Rhein Chemie Rheinau GmbH.
•
Dr. Gaston Aguilar, research director, R.T. Vanderbilt Co., Inc.
TLT asked these reps to address the issues and provide further guidance into using metal deactivators.
FUNCTIONS OF A METAL DEACTIVATOR
STLE-member Dr. Gaston Aguilar, research director for R.T. Vanderbilt Co., Inc., in Norwalk, Conn., says, “Metal deactivators are lubricant additives that counteract the catalytic effects of metals on oxidation and corrosion. The best understood mechanism for how they function involves the formation of inactive barrier films that interfere with the cathodic and/or anodic reactions that catalyze oxidation and/ or cause corrosion. By inhibiting corrosion, metal deactivators also prevent the formation of metal ions that catalyze oxidation.”
According to STLE-member Thomas Rossrucker, VP technology, and Dr. Sandra Horstmann, product specialist and application engineer-lubricant division for Rhein Chemie Rheinau GmbH in Mannheim, Germany, “Metal deactivators are surface-active molecules. They absorb on the metal surface, as shown in Figure 1. The polar part of the metal deactivator molecule is in direct contact with the metal surface, while the lipophilic part is on the opposite side interacting with the lubricant.”
Figure 1. Metal deactivators establish inactive barrier films through their polar part, which absorbs on the metal surface. On the opposite side, the lipophilic component interacts with the lubricant. (Courtesy of Rhein Chemie Rheinau GmbH)
STLE-member Dr. Ian Macpherson, senior marketing manager for Afton Chemical Corp. in Richmond, Va., provides further information on the role of the metal deactivator: “A metal deactivator prohibits or reduces the interaction between the metal surface and chemicals contained within the lubricant. The metal is often yellow (brass/bronze) though we have also dealt with silver from time to time. Besides forming a protective film, metal deactivators react with the chemicals in the lubricant that would otherwise tend to react with the metal surface.”
Metal deactivators react with dissolved metals to prevent them from degrading fuels and lubricants. Macpherson says, “Copper ions and many other nonferrous yellow metal ions act as reaction sites, speeding up auto-oxidation mechanisms in fuels and oils that lead to the formation of gums and deposits. Metal deactivator additives counteract the catalytic activity of these dissolved metals by sequestering them (i.e., hiding them).”
Derek Phillips, global commercial manager-metal protection for The Lubrizol Corp., in Wickliffe, Ohio, indicated that one of the key metals inhibited by metal deactivators is copper. He says, “One of the most common elements is copper, which when oxidized, may cause undesirable physical or chemical changes in strength or esthetic characteristics.”
Dr. Ron Pearson, R&D director for PMC Specialties Group, Inc., in Cincinnati, indicates that metal deactivators deliver a unique set of capabilities to diminish the potentially destructive impact of metal ions. He says, “The protective film they form on a metal surface is thermally stable, water insoluble and chemically bonded. A second important feature is that metal deactivators form very stable complexes through sequestering metal ions. The very large stability constants of these complexes indicate strong and practically irreversible binding with certain metals (
see Table 1) (
1). Of particular note is the copper complex, which has a stability constant of 10
15.
Table 1. Metal deactivators exhibit large stability constants with various metals, including copper. These large constants indicate that these metals bind with metal deactivators irreversibly. (Courtesy of PMC Specialties Group, Inc.)
STLE-member Pat Brutto, applications R&D leader for Dow Personal, Home & Industrial Care in Buffalo Grove, Ill., points out that metal deactivators will perform their function for only a specific time frame. He says, “A metal deactivator forms ionic or covalent bonds with the metal surface, reducing or preventing attack by the surrounding environment for a finite time.”
STLE-member Bridget Crocker, technical manager for PCC Chemax, Inc., in Piedmont, S.C., focuses on the use of metal deactivators in metalworking fluids. She says, “In water-extendable fluids, metal deactivators bind metal ions, which, in turn, will reduce the negative effects of water hardness. For straight-oil systems, they inhibit oxidative processes between the oil and the metallic parts of the system.”
KEY LUBRICANT APPLICATIONS
All of the respondents indicated that metal deactivators are used in most of the main automotive and industrial lubricants, including greases. Phillips says, “There is a very broad industry need for metal deactivators, especially where a lubricant is in contact with a transition metal or reactive metal substrate.”
Crocker points out that metal deactivators are needed in heavy-duty applications where active sulfur has to be used. She says, “The metal deactivator benzotriazole is a great example of an effective metal deactivator for copper and its alloys by preventing undesirable surface reactions.”
Metal deactivators also are used in applications where hard water is a problem. Crocker adds, “Metal deactivators, such as pentasodium DTPA (diethylenetriaminepentaacetate), prevent metal-ion reactions with fatty acids used in lubricants to minimize the formation of hard water soaps.”
Horstmann states that metal deactivators are widely used in almost all lubricants, as well as in anticorrosion oils/prelubes and in preservatives (solvent, oil or water-based).
Aguilar reveals that many metal deactivators are multifunctional additives that do not always have this role as their primary function. He says, “The metal deactivating effect of many well-known additives is overlooked or underestimated as an essential part of lubricant antioxidant systems. Classic examples of multifunctional additives with metal-deactivating properties are zinc dithiophosphates and zinc dithiocarbamates.”
In metal-removal fluids, metal deactivators also play a role during the manufacturing of tungsten-carbide tooling, according to Brutto. He says, “Metal deactivators, such as tolyltriazole, prevent the leaching of cobalt binder from the tool material.”
Macpherson also remarks that metal deactivators can be used in fuels. He says, “They play a critical role, particularly with jet fuels.”
SELECTION OF METAL DEACTIVATORS
Horstmann lists five specific criteria that the lubricant formulator must take into consideration in choosing a specific metal deactivator:
1.
The specific metal to be protected whether it is copper, ferrous or steel, bronze, aluminum alloys or zinc-coated steel.
2.
The basestock used in the application (mineral oil, water, PAO, etc.).
3.
The frequency of the application of the lubricant and whether it is always in contact with the protected metal part. In the application, does the metal deactivator need to be a film-former?
4.
The effectiveness of, and the length of time, the metal deactivator needs to be effective. One additional factor to be considered is the environment of the application.
5.
Additional properties needed from the metal deactivator, which may include demulsibility, emulsibility, color, metal-free, dewatering, toxicology, lubricity and compatibility with other additives.
Phillips points out that the chemistry of the metal deactivator is very important in determining its use in a specific formulation because of concern about how effectively it interacts with the metal surface and if it interferes with other additive types. He says, “Metal deactivators can be acidic, basic or neutral, so their interaction with the metal surface must be considered.”
Seth Crawley, technology manager for The Lubrizol Corp., indicates that compatibility with other additives is also important. He says, “A formulator must also consider what other additive technologies will be included in the final formulation such as detergency, antiwear and extreme pressure additives. These are all surface-active additives that compete for similar metal surfaces as the metal deactivators. The formulator must choose which property is most important for its particular application (for example, antiwear) and then choose a metal deactivator that does not interfere with that property.”
STLE-member David Lindsay, technical manager-metalworking fluid additives for Afton Chemical Corp., believes that cost and environmental considerations must also be factored into the formulator’s decision-making process. He says, “The U.S. Environmental Protection Agency, through its Design for Environment Initiative, is trying to encourage the use of sequestering agents/ chelators that have minimal environmental impact (and conversely trying to move formulators away from options that are considered to have a negative environmental impact).”
Crocker feels that there are three important factors to be considered in selecting a metal deactivator for a MWF: “the metal being machined, the water hardness found at the end-use application and the type of tooling involved in the machining process.”
Aguilar indicates that one other important factor to be evaluated is the temperature of the application.
ALUMINUM STAINING
Staining is most closely identified with MWF applications where a highly alkaline lubricant is machining a specific aluminum alloy. This problem can be very frustrating to resolve because of its variability. Light and dark-colored staining can be seen on aluminum and really reflects the aggressiveness of the process (
2). A darker color is reflective of more aggressive aluminum staining.
Brutto comments, “Staining is defined as a lightly corroded area generally confined to the surface and is typically caused by alkaline aqueous environments, which can disrupt the protective oxide layer. Discoloration and staining can also result due to optical effects associated with various aluminum oxides (
3). Substances as mild as tap water can stain aluminum, and this is made worse by additives such as amino alcohols.”
Brutto continues, “An industry study demonstrated that amino alcohol solutions create stains that increase at pH values above 8.0 (
4). In a MWF environment, approaches to minimize staining include: (1.) formulating at pH values below 9.0, (2.) minimizing use levels of amines and other alkaline materials, (3.) using soluble oils and high-oil semisynthetic fluids where the resulting emulsions help protect the aluminum and (4.) if necessary, adding materials such as phosphate salts/esters and/or sodium silicate to protect the metal surface.”
Crocker provides additional details on the cause of aluminum staining and ways to formulate around the problem. She says, “Aluminum is a very soft metal that reacts with oxygen in the air to form its own oxide coating. This self-made coating provides some protection at the metal surface against further staining. Unfortunately, this coating is not stable at pH values of 8.5 and above. These highly alkaline conditions break down the aluminum oxide coating, causing the staining.”
Crocker continues, “When formulating MWFs for aluminum machining, the formulator should target a pH between 8.5 and 8.8 to minimize staining. Appropriate metal deactivators such as phosphate esters are also recommended to control this problem when the pH needs to be higher than 8.8.”
Horstmann indicates that the cause of the aluminum staining could be due to either the presence of acids or bases. She says, “The aluminum oxide surface is destroyed by mechanical or chemical influences. Aluminum oxide is amphoteric, and bases or acids can attack the protective layer. This results in the formation of corrosion and leads to an uneven surface, which gives an impression of staining on the macroscopic scale. Other alloyed materials can also be involved and will react with different chemicals to generate colored products.”
To minimize aluminum staining, Horstmann says, “After machining, the cleaning of aluminum parts should take place as soon as possible to avoid accumulation of basic or acidic ingredients originating from the MWF.”
Lindsay discusses some guidelines for minimizing staining. He says, “Reducing staining is contingent upon knowledge of the metallurgy of the workpiece and the operating parameters for the process. Different aluminum alloys may require different approaches to reduce or eliminate staining. Generally, staining can be minimized through effective pH control and the correct selection of raw materials (e.g., amine selection). Additives such as silicates, phosphates and azoles may be used to suppress discoloration.”
Phillips says, “Aluminum staining is a big concern for the metalworking industry, and there is not one best approach. MWFs are much more complex and vary from formulator to formulator. Each MWF needs to be evaluated and modified in different ways. One of the most common methods of protecting aluminum from staining is with silicates or phosphates. Each may have its benefits and drawbacks, depending upon the application.”
SCREENING TESTS
For aluminum staining, Crocker recommends two simple tests that can be run in a lab to evaluate the efficacy of a metal deactivator. She says, “The first is a jar test where the substrate to be machined is half submerged in the test fluid containing a metal deactivator. A lid is placed loosely on the jar and the sample is kept in an oven for 24 to 48 hours at 40 C. The degree of staining on the substrate is then examined. If staining is seen, then an alternative metal deactivator is needed for the application.”
Figure 2 shows a series of aluminum alloys evaluated by the jar test for staining. On the left are those test coupons that have stained, while the ones on the right remained free of staining.
Figure 2. The jar test is used to evaluate aluminum alloy test coupons. Those on the left have stained, while the ones on the right remained free of staining. (Courtesy of PCC Chemax, Inc.)
Crocker continues, “The second test is the IP 263 emulsion stability procedure for water-extendable fluids. This test is used if hard water is a problem. The continuing presence of hard water soaps after 24 hours means that the incorrect metal deactivator is being used.”
For more precise results, Crocker suggests that the metal substrate be evaluated for weight loss or a technique such as atomic absorption spectroscopy should be used to measure the concentration of metal ions in the MWF.
Brutto comments that testing of aluminum alloys (and staining inhibitors) should encompass exposure to both liquid and vapor phases of the fluid environment. He says, “As always, field conditions should be duplicated as best as possible (temperature, water source, dilution rate, etc.). Exposure of as-supplied coupons, as well as freshly abraded ones (to expose metal under the oxide) should be standard practice in lab evaluations.”
Aguilar agrees that simple exposure tests using metal specimens under controlled temperature and time conditions are used to evaluate metal deactivators. He says, “For industrial oil applications, the test methods commonly referred to as copper strip tests are ASTM D130 for oils and ASTM D4048 for greases. Bulk oxidation test methods that include the use of a metal catalyst are also very useful. Examples of well-known standardized test methods are the turbine oil oxidation tests such as ASTM D943 and ASTM D4310, the Rotating Pressure Oxidation Test (RPVOT, ASTM D2272) and the Cincinnati Thermal Oxidation Stability Test.”
The ASTM D130 test is typically run by immersing the copper strip in a test fluid for three hours at 100 C. Once the test is completed, the copper strip is rated based on a color scale ranging from 1 to 4. Table 2 lists the ASTM classifications and the resulting descriptions.
Table 2. The copper strip classifications used in ASTM D130 are shown. (Courtesy of ASTM)
Figure 3 shows two copper strips run under ASTM D130 with and without metal deactivator. The control on top contains no metal deactivator and has a rating of 4b, while the copper strip with metal deactivator contains no evidence of stain and has a rating of 1a.
Figure 3. Two copper strips were evaluated in lubricants with and without metal deactivator using ASTM D130. The control on top contains no metal deactivator and has a rating of 4b. The strip on the bottom was used in a lubricant with metal deactivator and has a rating of 1a, indicating no evidence of stain. (Courtesy of PCC Chemax, Inc.)
Crawley pointed out that while copper corrosion testing is a key test, evaluating the impact of the metal deactivator on ferrous corrosion is also important. He says, “ASTM D665/ISO 7120 is effective for assessing steel corrosion inhibition.”
Horstmann feels that there are many screening tests available for the formulator to use. Selection of the proper tests is dependent on the metal to be protected and more or less on the properties needed for the application. She says, “Typical tests for ferrous metals are the steel finger corrosion test (ASTM D665) and various storage tests at high humidity or in a saline environment (e.g., salt spray test ASTM B117), the alternating atmosphere test [DIN EN ISO 6270-2], for yellow metals the copper strip test (ASTM D130) and for other metals, storage tests in the individual lubricant.”
Besides the tests already discussed, Macpherson lists several other procedures that should be used including alkalinity, acid value, foam generating potential and stability.
DIFFERENTIATE PERFORMANCE OF INDIVIDUAL METAL DEACTIVATORS
Aguilar stresses the need to look at temperature as a means to determine how one metal deactivator performs versus another. He says, “There are two basic mechanisms for barrier-forming metal deactivators. They either build a passivation barrier by strongly and directly absorbing to the metal surface or they can react with the metal surface and/or metal ions to deposit a protective film. This latter process is known as conversion layer formation.”
Aguilar continues, “For low-temperature lubricant applications, the direct adsorption mechanism is more effective. The opposite is true for moderately high temperature applications. At high temperatures, the trend is reversed again since metal deactivators that form conversion layers tend to decompose to more corrosive acidic materials. This phenomenon is particularly true when the metal deactivator is formulated with phosphorus and sulfur-based compounds.”
Phillips cautions that metal deactivators consist of different chemistries. He says, “The formulator must evaluate each chemistry to determine applicable performance and compatibility.”
Crawley stresses the importance of formulation stability. He says, “Storage stability must also be considered, as metal deactivators can react with other additives in the formulation during storage. Furthermore, metal deactivators also can affect the results of other screening tests including ASTM D4310/ISO 4263-1, ASTM D2070 (Cincinnati Thermal Stability) and others.”
From the MWF standpoint, Crocker indicates several parameters to look for in differentiating metal deactivators. She says, “The formulator should assess the amount of staining, the weight loss of substrate or the presence of hard water soaps formed. Metal deactivators need to be evaluated under different conditions (i.e., ambient temperature and elevated temperatures).”
INTERACTION WITH OTHER LUBRICANT ADDITIVES
Horstmann believes the formulator can minimize potential problems between metal deactivators and other lubricant additives by determining whether a metal-containing or ashless material is used. Very often acid-base type reactions take place. “Therefore, one should avoid combinations of basic performance additives with acidic metal deactivators and should use instead combinations of similar acidity/ basicity,” She says. “The chemical structure of the different additives influences whether they will interact synergistically or antagonistically. As a basic rule, the formulator should combine metal-containing additives with metal-containing additives and ashless with ashless additives. This will avoid most of the negative effects.”
Macpherson cites potential concerns with formulating specific metal deactivators with metal-containing additives. He says, “Thiadiazoles are designed to react with other additives. Occasionally we have seen negative interactions with metal-containing additives. The triazoles are sometimes a challenge to dissolve in either the package or the finished oil.”
Lindsay adds, “Solubility in oil is important, and the stability of the product in water are important in MWFs.”
Crawley cited that whether the metal deactivator is acidic, basic or neutral is important when considering whether it can be used in a lubricant with minimal problems. He says, “The chemistry of the metal deactivator will determine if it will interact with other additives. This means that selection of additives, in general, and metal deactivators, in particular, for a specific lubricant must be done carefully. Basic metal deactivators can react with acidic additives (and vice versa). Metal deactivators that are neutral (neither acidic nor basic) may be less likely to negatively interact with other additives.”
Aguilar points out those metal deactivators can work synergistically with other lubricant additives such as antioxidants and extreme pressure agents. He says, “Metal deactivators that adsorb directly on metal surfaces are typically benign and do not interfere with other additives at loads, speeds and temperatures seen at tribological contacts. They also work very effectively with antioxidants that function by other inhibiting mechanisms such as radical scavenging and peroxide decompositions. Triazoles such as benzotriazole, tolyltriazole and their derivatives are examples.”
Aguilar continues, “We combined a tolyltriazole derivative with two ashless components (an ashless dithiocarbamate that provides antioxidation, extreme pressure and antiwear properties and an ashless rust inhibitor) in a Group I base oil. Figure 4 shows a major improvement in antioxidation performance from results obtained with the Rotary Pressure Vessel Oxidation Test (ASTM D2272). Specifically, the data shows 350 to 700 ppm of the tolyltriazole derivative vastly improved the antioxidant capacity of the dithiocarbamate, most likely through deactivation of the copper in the test.”
Figure 4. RPVOT data for Group I base oils treated with variable proportions of an ashless dithiocarbamate and tolyltriazole derivative with all formulations containing a total of 0.7 mass percent of these two additives. All formulations contain 0.1 mass percent of succinic half-ester rust inhibitor. (Courtesy of R.T. Vanderbilt Co., Inc.)
Metal deactivators that function by the conversion layer mechanism act differently as compared to those that act by direct adsorption. Aguilar explains, “Metal deactivators that function by the conversion layer mechanism are typically multifunctional, and, thus their interactions with other additives are much more complex for formulators trying to balance multiple performance parameters at a time.”
Rossrucker and Horstmann agree that metal deactivators can improve other properties of a lubricant by deactivating the catalytic effect of metal ions. Additional data (
see Figure 5) is presented showing how a common metal deactivator, tolyltriazole, can improve the performance of two antioxidants in the RPVOT Test (ASTM D2070).
Figure 5. A small treat rate (0.02%) of tolyltriazole (TTZ) improves the performance of an antioxidant (AO) and the phenolic antioxidant, butylated hydroxytoluene (BHT) in the RPVOT Test. (Courtesy of Rhein Chemie Rheinau GmbH)
FUTURE TRENDS
Four of the respondents cited regulatory trends as important in the development and use of metal deactivators in the future. Macpherson says, “Use of metal deactivators will be influenced in the U.S. by the EPA as the Design for the Environment initiative continues to be pushed.”
www.canstockphoto.com
Horstmann discusses the need for formaldehyde-free metal deactivators. She says, “Non-toxic metal deactivators (e.g., formaldehyde-free products) will be needed in the marketplace as formaldehyde derivatives are eliminated. Improved solubility of metal deactivators in nonpolar solvents (such as Group II, Group III base oils and PAO) will also be important as their use grows.”
Phillips looks at the future use of metal deactivators in MWFs. He says, “As the metalworking industry increases the use of transition and reactive metals inside equipment and metal that is processed, additives with better toxicity profiles, improved thermal and hydrolytic stability are needed.”
Crawley indicates that there are similar trends for other lubricants. He says, “The toxicological and environmental impact of metal deactivators will continue to be important. Also, metal deactivators that offer thermal stability and compatibility with other additives will be sought.”
Aguilar stresses that new challenges will be faced by metal deactivator suppliers when new surface coatings are developed that alter the properties of metals. He says, “However, it is too early to tell what coating technologies will come to the forefront and which surface properties such as coefficient of friction, corrosion and wear resistance will be improved by these coatings or which properties will need improvement from formulated lubricants.”
The growing use of nonferrous alloys in lubrication systems will necessitate the greater need of metal deactivators to protect against premature deterioration. Lubricant additive suppliers will assist formulators either through the development of new metal deactivators or multifunctional additives whose performance profile includes the desired properties of metal deactivators.
REFERENCES
1.
Hernandez, M. and Abu-Dalo, M. (2008), “Removing Metals from Solution Using Metal Binding Compounds and Sorbents Therefor,” U.S. Patent 7,361,279 B2.
2.
Becket, G. (2006), “Corrosion: Causes and Cures,” in Byers, J.,
Metalworking Fluids Second Edition, CRC Press, pp. 177-179.
3.
Shreir, L., Jarman, R. and Burstein, G. (1994), “Non-Ferrous Metals and Alloys,” in
Corrosion Third Edition, Volume 1, Section 4.22.
4.
(2003), “Amine Selection for Metalworking Applications,” TLT,
59 (9), pp. 24-26.
Neil Canter heads his own consulting company, Chemical Solutions, in Willow Grove, Pa. Ideas for Tech Beat items can be sent to him at neilcanter@comcast.net.