20 Minutes With Chris Napoleon
Karl M. Phipps, Managing Editor | TLT 20 Minutes December 2011
This new-product specialist develops inspection and testing programs to evaluate bearing quality.
CHRIS NAPOLEON - The Quick File
Chris napoleon is the president and chief engineer for napoleon engineering services, Olean, N.Y. founded in 1997, NES specializes in bearing inspection, bearing testing and custom-bearing manufacturing. Involved in bearing design, failure analysis, bearing qualification inspection and dynamic test programs for some of the largest original equipment manufacturers in the U.S. and around the world. His custom-bearing manufacturing facility specializes in supporting bearing manufacturers, distributors, OEMs and end-users with custom designed and manufactured bearing products that meet the needs of challenging application conditions, with a focus on the aerospace industry. In addition, he drives the development of bearing technology and manufacturing excellence for advancements in product, process and application capabilities.
Work Experience
Chris has more than 21 years of experience working in the bearings industry specializing in rolling element bearings. Previously worked in the field of bearing manufacturing, design and testing for SKF Aeroengine (formerly MRC bearings) responsible for manufacturing engineering and advance manufacturing planning, application engineering, new product development and testing. He has traveled around the world working with and auditing bearing manufacturing facilities. He is internationally known as a specialist in the field of bearing qualification practices.
Education
• Master’s of Science, Manufacturing Management – Kettering University, Flint, Mich.
• Bachelor’s of Science, Mechanical Engineering – University of Pittsburgh, Pittsburgh, pa.
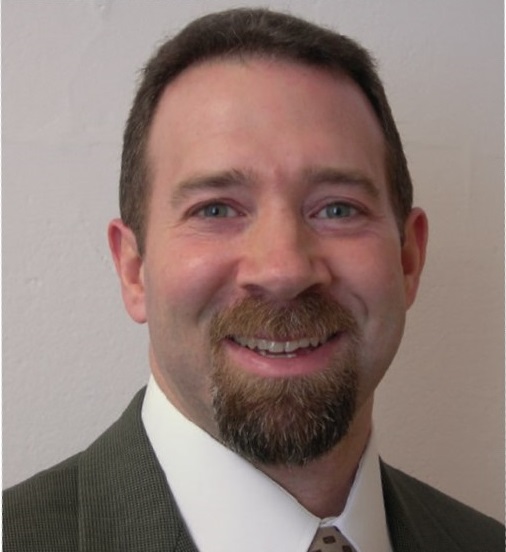
Chris Napoleon
TLT: What was the driving force that influenced you to develop programs for evaluating bearing quality?
Napoleon: I started Napoleon Engineering Services in 1997 to pursue bearing inspection, bearing testing and custom-bearing manufacturing. Because of my previous experience in the aerospace bearing industry, I became involved in reverse engineering of type-certified aircraft bearings in support of a customer’s desire to achieve parts manufacturing approval (PMA).
The PMA process is a detailed inspection of all characteristics to determine how and why the bearing performs successfully in the application. I would perform a complete dimensional, chemical and material analysis. The PMA process has been in use for decades and is a proven process for qualifying new sources of supply. In addition to PMA reverse engineering, I was involved in a large quantity of bearing failure analysis work for OEMs. I also was being sought for bearing inspection by OEMs and bearing distributors who were interested in sourcing bearing products directly from the global supply chain. From a business standpoint, there were not a lot of independent bearing inspection facilities available that were interested in supporting the needs of industrial OEMs for bearing inspection and qualification.
From a technical perspective, I noticed that a large percentage of the failure analysis work was coming from OEMs that had recently changed their source of supply, having not performed any specific qualification program. As a result, OEMs now wanted me to determine why the bearing in their time-proven application was failing prematurely. On the aircraft side, the industry was following a proactive approach of reverse engineering qualified bearings to define those design and manufacturing characteristics that were proven successful in the application in support of qualifying a new source of supply. However, industrial bearing users were taking a reactive approach to the problems occurring from using a new source. So I developed the Source Qualification Inspection (SQI) program, which is essentially the industrial version of the FAA-approved PMA reverse engineering program.
I found that most OEM design engineers did not know why the original supplier or baseline bearing worked in their application—they only knew that it did. Perhaps at some point they had performed lab or field testing and the bearing passed. Unfortunately they did not know why it passed. They lacked any real knowledge of the internal design characteristics and the manufacturing plant’s ability to carry out the design intentions to assign cause to its success. The deeper you go in bearing inspection, from dimensional characteristics, noise quality, visual inspection to chemical and metallurgical characteristics, the more you uncover the power of bearing inspection as a real qualification tool. This is no mystery for most reputable bearing manufacturers, but for OEMs it’s really a new tool for them.
TLT: With the global supply chain of bearing products today, can bearings be considered a commodity?
Napoleon: I have over a decades worth of inspection and testing data showing that not all bearings are created equal, even from the same source of supply. There are two reasons: (1.) the quality standards governing the design of a bearing are minimal, leaving plenty of opportunity for creativity. That is not necessarily bad until you compare a world-class bearing manufacturer with substantial research and development behind their product to a manufacturer who lacks those resources and (2.) the large quantity of acquisitions and joint ventures taking place in the industry, primarily by the large bearing manufacturers looking to establish a global manufacturing presence. I regularly find different design and manufacturing characteristics from a manufacturer who has multiple plants around the world. However, this does not mean that a successful global supply base for bearings cannot be achieved. It just requires the use of a qualification program that provides the correct amount of risk reduction.
If you consider the internal design of a tapered roller bearing, for example, it possesses several very important rolling and sliding contact surfaces that require evaluation. The cone raceway, cup raceway and roller OD contours play a major role in reducing roller end stresses and the bearing’s ability to adequately support misalignment in the application. In addition to raceway contour or profile magnitude, there are different forms created by the manufacturing process that can be compared and analyzed (
see Figure 1). When a tapered roller bearing operates under a radial load, there is a resultant axial load that drives the roller ends into the cone sidewall or rib flange. The contours and surface finish values of these mating surfaces play a major role in bearing heat generation, as well as break-in characteristics.
Figure 1.
Differences in surface finish and relationships between roller end and rib flange profiles can significantly affect the lubricant characteristics. The original oil supply requirements might no longer be adequate, resulting in premature bearing failure. Raceway roundness and harmonic analysis of the raceway circumference provides an indication of the noise signature the bearing will have (
see Figure 2). These are just a few characteristics that need to be examined to adequately judge a bearing’s quality level. It’s easy to see that differences in bearing design and manufacturing quality create significant risk to an OEM if they consider all bearings as a commodity.
Figure 2.
TLT: How has the role of an OEM design engineer changed relative to specifying bearings?
Napoleon: Bearings cannot always be considered commodities. Therefore, just defining an industry part number is not sufficient to ensure acceptability of a new bearing supplier’s product in many applications. I’ve found that the drawings that OEMs have depicting the requirements for the bearing are often inadequate in defining the necessary characteristics to ensure success when sourcing globally.
In my opinion, I believe OEM design engineers can do several things to mitigate their risks when specifying bearings. Documenting the characteristics of the baseline bearing that currently works in the application through the use of an extensive inspection program is a good starting point. From that information, the OEM drawings can be populated with specific characteristics that will reduce the variability in design differences. They also need to evaluate the criticality of the bearing in the application and consider developing a dynamic test plan.
In addition, the design engineer should document what the typical failure modes are and determine their specific needs or intentions for testing to offset failures in these areas. From that information, a test plan can be proposed. If the failure mode is fatigue failure or if warranty costs could be catastrophic from premature fatigue failure, then a rolling contact fatigue test should be conducted. We might be comparing what the combined effects of design, manufacturing and material quality have on the empirical life of one supplier versus another. Most often, a sudden death, least-in-four, test program is used. A typical test rig would allow for radial, axial and possibly moment loading to be applied to four bearings at some predetermined speed and lubrication condition (
see photo).
Chris Napoleon working on a test rig at his bearing test lab.
The bearings are run until one bearing in the group experiences fatigue failure. Then the test rig is rebuilt with four new bearings and again run until fatigue failure occurs. This sequence is repeated until an adequate number of failure data points is accumulated. The testing can be performed on any number of suppliers. Emperical L10 life values are determined from the Weibull analysis, allowing for a comparison to the calculated theoretical life based on the test conditions. This information is invaluable to the design engineer and often imperative to risk mitigation when global sources are considered for critical applications (
see Figure 3).
Figure 3.
TLT: How valuable are bearing inspection and testing programs to the designer engineer?
Napoleon: The use of inspection and testing programs to support bearing qualification programs is most valuable to the design engineer when used in combination. I see little value of performing a dynamic test plan if you don’t know what you’re testing. The addition of a solid inspection of a sample population of product before the dynamic portion of the qualification process serves two purposes. First, if the inspection determines that the product possesses significant deficiencies in design, manufacturing or quality of workmanship, there may be no need to perform dynamic testing until the issues are addressed and corrected, saving valuable time and money on dynamic testing. Second, if dynamic testing shows one supplier to be significantly better than the another, the addition of the inspection data in the analysis phase may allow the designer to assign cause to or explain the difference in the test results. Again, specific changes can now be made and product retested that should result in improved bearing life and qualification of a new source of supply.
During my experience, there are five key factors required for successful utilization of global bearing suppliers:
1.
Having a good understanding of one’s application and the failure modes associated with it. Having warranty data on the bearing is also helpful.
2.
Defining the criticality of the bearing within the application.
3.
Using detailed inspection programs for documentation of baseline and new bearing supplier characteristics. Creating detailed OEM drawings based on the data.
4.
Performance lab or field testing for empirical life comparisons.
5.
Top-down commitment in time and money focusing on improving in-house bearing knowledge through long-term bearing testing programs.
It certainly takes more work on the part of the OEM than in past years to qualify a bearing supplier. However, if performed correctly there are significant benefits to be gained in having a better control of your own destiny regarding product availability and cost.
You can reach Chris Napoleon at cnapoleon@nesbearings.com.