Microbial problems in metalworking fluids
Dr. Frederick J. Passman | TLT Cover Story August 2009
Understanding four significant factors can help control microbial contamination in metalworking operations.
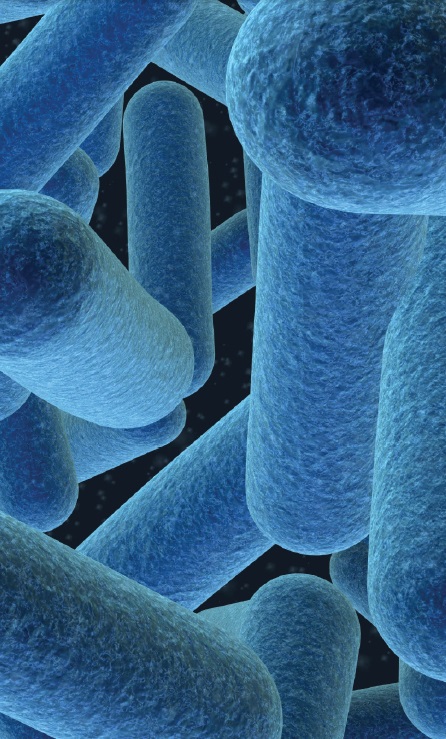
www.canstockphoto.com
KEY CONCEPTS
•
By understanding basic concepts about microbial life, a plant manager can take effective measures to monitor and control microbial contamination.
•
Four factors dominate in controlling microbial life: energy source, nutrients and acceptable thermal and pH conditions.
•
Metalworking fluids are complex mixtures of chemicals that are constantly changing due to the chemical, physical and microbiological factors which typify metalworking systems.
Author ’s Preface
I am honored that TLT feels that my 1988 article, “Starting from Scratch: Microbial Problems in Metalworking Fluids,” deserves a third printing. I added a postscript to the 2004 reprint and offer this preface to the current edition.
Most of my current thinking on MWF microbiology is captured in Chapter 9 of the 2006 book
Metalworking Fluids, Second Edition, edited by Jerry Byers and available at a discount to STLE members at
www.stle.org.
Additionally, the past five years have been very productive ones for ASTM E.34.50 Health and Safety on Metalworking Fluids. In the past two years, we have developed four new Test Methods that provide important tools for improved fluid condition monitoring and health risk assessment:
E2563: Enumeration of Non-Tuberculosis Mycobacteria in Aqueous Metalworking Fluids by Plate Count Method
E2564: Enumeration of Mycobacteria in Metalworking Fluids by Direct Microscopic Counting (DMC) Method
E2647: Endotoxin Concentration in Water-Miscible Metalworking Fluids
E2694: Determination of Adenosine Triphosphate (ATP) in Water-Miscible Metalworking Fluids.
There’s still much that we don’t know, but in 2008 we launched the fledgling STLE MWF Microbiology Interest group. I hope this group will promote collaboration among companies to better understand the ecology of microbes in MWF systems. Better understanding the ecology enables us to reduce both the health and biodeterioration risks caused by MWF microbe.
Finally, since 2004 both the Biocidal Products Directive and REACh have been implemented. It’s likely that the toxicological data required under REACh will be very similar to that currently required for MWF microbicides. Consequently, the economic impetus for companies selling unregistered biocides as “biostable additives” is likely to disappear.
Dr. Fred Passman
August 2009
All metalworking fluid formulations share the common problem of susceptibility to microbial attack. This is not all bad news, since we need the used dilute fluid to be biodegradable for disposal purposes.
However, the challenge for both formulators and metalworking facility operators using water-based fluids is minimizing the adverse economic impact of uncontrolled microbial contamination in metalworking operations. The following discussion focuses on water-extended fluids. A short glossary is provided in Table 1 to assist the reader who is unfamiliar with the microbiological terms used in this article.
Table 1. Glossary of Microbiological Terms for Metalworking
Aerobe: A microorganism that can only grow when adequate oxygen is present. Oxygen serves as the terminal electron acceptor.
Anaerobe: A microorganism that can only grow in the absence of oxygen. Nitrate, sulfate or organic compounds serve as terminal electron acceptors in different species.
Bacterium: A single-cell organism. Bacteria are the smallest living units capable of carrying out, independently, all of the basic functions that characterize living beings (respiration, growth, assimilation, metabolism, excretion and reproduction). Bacteria may be as small as 0.1 μm (4 x 10
–6 in.) in diameter and may appear as spheres, rods, commas or spirals under a microscope. As a kingdom, the bacteria represent a vast diversity of nutritional and survival capabilities.
Biocide: A chemical that kills living organisms.
Biofilm: Complex layer comprised of microorganisms and their secretions as well as detritus trapped within the biofilm matrix. Biofilm thickness may range from a few microns to several centimeters. Physical and chemical conditions within biofilms are controlled by the microorganisms growing there and may be very different from conditions in the bulk fluid. Many species of microorganisms form the biofilm community.
Biomass: The total amount of living organisms in a given volume of material. Difficult to measure directly, biomass is generally extrapolated from the measurement of a chemical component of the living cells within the mass. Optimally, the average concentration (per cell) of the chemical being measured is either known or a standard conversion factor has been established.
Biostat: A chemical substance that prevents the growth or proliferation of living organisms but does not necessarily prevent metabolism.
Colony: The mass formed on the surface or within the matrix of microbial growth media as a result of the reproduction of ostensibly one cell. A bacterium with a generation time of 1 hour (population doubles each hour), will form a colony containing over 2 billion cells in about 30 hours. The colony, visible to the naked eye, is easier to count than individual microbes. Problems arise because not all microorganisms grow on the same nutrients or under the same conditions. Bacteria and fungi grow in a coolant often fail to form colonies on solid growth media.
Corrosion-enhancing microbial activities: Some bacteria, like the sulfate-reducing bacteria, contain the enzyme “hydrogenase,” which scavenges hydrogen ions and creates a galvanic cell. Many microbes manufacture organic acids which attack metal surfaces directly. The very presence of nonuniform biofilms causes electropotential gradients to develop. All of these activities tend to accelerate corrosion rates.
Detritus: Unwanted particles floating on the surface, suspended in the bulk mass or precipitated out to the bottom of metalworking fluid. Swarf, flocs of biomass, detached rust deposit fragments all make up typical detritus in coolant systems.
Dip stick/dip slide: One of a number of paddle-like devices either coated or saturated with a growth medium. They are dipped into the coolant to be tested, incubated for 1-2 days and observed for the development of colonies. They provide a simple means of getting very approximate plate count data.
Disinfect: To destroy or inactivate harmful bacteria.
Not equivalent to sterilization.
Electron-acceptor molecule: A molecule like oxygen that captures electrons and becomes reduced to water. All cells derive their energy from a sequence of reactions involving the transfer of electrons along a “cascade” of molecules. The last molecule in this sequence is the
terminal electron acceptor. For aerobic organisms, oxygen is the terminal electron acceptor. Sulfate serves this function for sulfate-reducing bacteria.
Enzyme: A molecule or cluster of molecules composed of long chains of amino acids. The enzymes are the cell’s metabolic factories. They act as catalysts for the metabolic reactions of all biochemical pathways. Active enzymes can cause fluids to become rancid even though the cells may be judged “dead” by some other criterion, such as plate counts.
Essential elements: Carbon, hydrogen, oxygen, phosphorous and sulfur are the elements without which life, as we know it cannot exist. All of these elements are present in abundance in metalworking fluids.
Facultative anaerobe: A bacterium that lives like an aerobe when oxygen is present, and like an anaerobe when oxygen is absent. Facultative anaerobes play a key role in creating and maintaining environments in which anaerobes can grow.
Fungicide: A chemical that preferentially or selectively kills fungi.
Fungus: The simplest microorganisms having a true cell wall. They appear as single-cell yeasts (approximately 10,000 times the volume of most bacteria) or as filamentous “molds.” These filaments are long strands (hyphae) of cells which form the fibrous network of growth one sees when looking at moldy food. The colored bodies found in a filamentous mat contain spores. One of these bodies may contain several hundred spores, each of which can give rise to a fungal colony.
Growth: Growth is the measurable increase of an individual’s or population’s biomass. See definition of “biomass.”
Growth rate: The amount by which the biomass increases per unit period of time (usually hours). Often growth rate is reported as the amount of time it takes for the population to double, assuming that biomass per organism is constant.
Inhibition: The prevention of any particular activity. Corrosion inhibition, microbial growth inhibition and foam inhibition are examples of inhibiting functions required of metalworking fluids. Inhibition is rarely absolute, so cost-benefit ratios should be computed to determine the merits of various inhibitor products.
Metabolism: The enzymatic reactions by which cells break down food sources (anabolism) and create new cell material (catabolism), giving off heat and waste “metabolites” in the process.
Microbially-mediated process: Processes such as corrosion and pH drop which are the direct or indirect result of microbial activity. For example, bacteria secrete organic acids which react with pH buffers, leading to a loss of pH control. The consequent drop in pH is microbially-mediated.
Microbicide: (Often written “microbiocide”) is an agent which is designed to kill microorganisms, ie., both bacteria and fungi.
Mold: See “Fungus.”
Nutrient: Any substance that an organism needs in order to grow and proliferate. In order for a chemical to function as a nutrient, the organism must be able to assimilate it (bring it into contact with the appropriate enzymatic machinery). Nutrients are “essential” if an organism cannot survive without them. “Non-essential” nutrients are important for healthy growth but not for survival.
Pasteurize: To heat-treat a fluid (usually with steam) in order to kill-off potentially pathogenic microorganisms. Pasteurization requires exposure to 61-63 C (142-145 F) for 30 minutes.
Note: many non-pathogenic microorganisms generally survive pasteurization.
Plate counts: A standard method for enumerating bacteria and fungi. A small sample portion is spread onto the surface of a suitable nutrient-containing gel. After incubation, colonies develop. (see “Dip-sticks”) This traditional technique is called the “plate count” because the nutrient gel is generally contained in a standard 100 mm “petri dish” or “plate.” Like dipstick methods, microbes must grow on the nutrients provided to form visible colonies. Consequently, these methods are often referred to as “viable counts.”
Proliferation: The increase in the number of individuals in a population. It may or may not be proportional to growth: for example, if cells are dividing, but there is no net biomass increase, the population is proliferating but not growing. Often, in metalworking systems, biomass increases are not accompanied by increased cell numbers. If viable counts are the only measurements of microbial contamination being used, the data may be dangerously misleading.
Sterilize: The complete destruction of biological activity.
Yeast: See “Fungus.”
Metalworking fluid spoilage can be defined as any change in the fluid that adversely affects its utility. Table 2 lists the most common indications of spoilage in metalworking fluids.
Table 2.
When any of these listed problems begins to occur with a coolant that has previously performed well, uncontrolled microbial contamination should be suspected and corrective action taken.
In order to determine what action is appropriate, a manager must have a feel for the fundamental nature of the problem. We will discuss some of the more salient principles of metalworking fluid microbiology.
Metalworking fluids become rancid because they contain rich blends of nutrients that encourage microbial growth. Mineral oil basestocks, glycols, fatty acid soaps, amines and other nearly universal constituents of metalworking fluid concentrates provide all of the essential nutrients required for growth. Not all fluids contain the same nutrients nor are they used under identical conditions. Consequently, the types of microbial contaminants which dominate may vary.
TYPES OF MICROORGANISMS
Two types of microorganisms contaminate metalworking fluids: bacteria and fungi. Bacteria are single-celled microorganisms lacking a true cell wall. Unlike higher organisms, their shape and nutritional requirements often reflect the environment from which they are recovered, rather than their genetic makeup. Consequently, a broad spectrum of genetic, physiological and morphological tests are generally needed for identifying a given bacterium. Gross classification often can be accomplished with as few as eight tests, but complete identification may require more than 300 tests.
In contrast to bacteria, fungi have true cell walls, like the cells of all higher plants and animals. In coolant systems, fungi may appear as single-celled yeasts or as filamentous molds. Many fungi spend part of their lifecycle as yeasts and part as molds. The factors, which determine whether a fungus will propagate in one form or the other are beyond the scope of this discussion. Some of the bacteria and fungi most commonly found in metalworking fluids are listed in Table 3.
Table 3.
In reality, the specific identity of a bacterial or fungal contaminant is of limited practical value in managing contamination problems. It is much more valuable to know:
•
What are the contaminants doing to the system?
•
What can be done to prevent that from happening?
UNDERSTANDING MICROBIAL LIFE
By understanding a few basic concepts about microbial life, the plant manager can take effective measures to monitor microbial contamination and control it intelligently.
Microbiologists concerned with spoilage prevention try to define microbial activities. Populations can be defined by their impact on the coolant or system. Table 4 lists some microbial activities that can be monitored in metalworking fluids and methods used to measure these activities. Gross observations of the phenomena listed in Table 2 are also important for monitoring microbial activity. Control is achieved by taking measures that minimize the rates at which these microbially mediated adverse processes occur.
Table 4.
Four factors dominate in controlling microbial life: an energy source, nutrients and acceptable thermal and pH conditions.
ENERGY SOURCE
Energy comes either from light, as for photosynthetic microbes and plants, or from the breakdown of oxidized organic molecules. The pathways involved in energy metabolism require molecules such as oxygen, sulfate or specialized oxidized organic molecules that can act as Lewis acids (i.e., molecules tending to accept electrons in chemical reaction) to drive the process along. These molecules accept the electron liberated at the end of a cascading series of oxidation-reduction reactions and are consequently called terminal electron acceptors.
Cells of all higher life forms (including the fungi) use oxygen as their terminal electron acceptor. Aerobic bacteria require oxygen for energy metabolism. However, anaerobic bacteria cannot grow in the presence of oxygen. Some species use sulfate, others use nitrate, but most anaerobes use “high energy” organic molecules as their terminal electron acceptors. The four-carbon organic acid, fumarate, is one such molecule.
Facultative anaerobes grow whether or not oxygen is present. When oxygen is present, they use it just as other aerobes do. When oxygen becomes depleted, they shift to an anaerobic mode of metabolism. Thus, they play a critical role in creating hospitable environments for odor and corrosion-enhancing microbial activities, which occur principally under oxygen-free conditions.
NUTRIENTS
Organic compounds and mineral salts present in metalworking-fluid formulations and makeup water are listed in Table 5 and were discussed above. Besides serving as nutrients, inorganic salts appear to make microbial populations more resistant to biocide treatment.
Table 5.
Typically, growth rate and metabolic activity increase as temperature increases, up to a point. Living microbes have been recovered from Arctic ice and from deep-sea vents where temperatures exceed 120 C (248 F). Further temperature increases inactivate enzymes and cause rapid die off.
The temperature at which die off occurs is species-specific. Thus, pasteurization (brief exposure to 61 C-63 C (142 F-145 F) inactivates most common pathogens, but prolonged super-heated steam treatment—say 15 minutes at 121 C (250 F) is required to kill many of the species commonly recovered from metalworking fluids. Despite brief exposure to extreme temperatures at the tool-workpiece interface, metalworking fluids do not normally achieve bulk temperatures sufficient to control microbial growth. In fact, in most systems the bulk-fluid temperatures are optimal for growth.
INTERVIEW WITH THE AUTHOR
TLT: Your name has become synonymous with research in MWF microbiology. How did you get your start?
Passman: My interest in environmental microbiology started when my parents gave me a microscope as a birthday present when I was eight years old. We spent our summers in Atlantic City, so the first samples I collected were seawater.
As an undergraduate at Indiana University, I had an opportunity to do several independent research projects, including an investigation of microbes growing in Southern Indiana cave streams. After my active-duty Naval service, I completed my doctoral studies in marine microbiology at the University of New Hampshire.
In 1978, while working on a dive project off of some Gulf of Mexico oil platforms, I met Ed Bennett. One of Ed’s University of Houston colleagues was running organic chemistry tests on our samples. At the time I was the microbiology lab director at Energy Resources Co. (ERCO) in Cambridge, Mass. When we moved ERCO’s microbiology group to Houston in 1981, Ed hosted my team for several months—permitting them to work in his lab—while we waited for our lab furniture to arrive. Subsequently, he referred a number of metalworking fluid industry clients to me and convinced me to get involved with STLE.
While ERCO’s microbiology team was still in Cambridge, our engineering consulting group asked me to evaluate a variety of oilfield biocides. The oilfield biocide work, coupled with the consulting work that I was doing for a growing number of metalworking fluid end-users, set the course of my activities ever since. The common theme has been the application of simple, rapid field tests that non-technical personnel can use to measure microbial contamination and assess microbicide performance.
In April 2009, two new ASTM methods under the oversight of ASTM Subcommittee E.34.50 (Health and Safety of MWF) were approved: E2657
Endotoxin in MWF and E2694
Adenosine Triphosphate (ATP) in MWF. I then recalled my marine work on endotoxin and ATP as indicators of total biomass, which led to me becoming a member of ASTM in 1978.
I met Harold (Hal) Rossmoore in 1983, when he, Ed Bennett and I were all on the same program at an SME workshop. Over the years, Hal drafted me to become an Associate Editor for Lubrication Engineering (TLT’s predecessor), and co-course chair for the STLE Basic Metalworking Fluids Course. Both Hal and Ed were very influential in my STLE involvement and my transition from marine microbiology to biodeterioration control.
TLT: What was the path that led you to chair STLE’s Metalworking Fluid Steering Committee and the society’s newly formed certification board?
Passman: In 1996, when I became co-chair of the metalworking fluid course, Tom Payne was retiring Education Course Committee Chair. Tom recruited Phil Guichelaar to succeed him and me to be vice chair. That started the sequence of my following Phil though the Course Committee and Education Committee chairmanships.
In 2003 John Burke asked me to participate in the ad hoc committee that recommended STLE to develop a metalworking fluid management certification (CMFS™) program. After STLE adopted the committee’s recommendation, I became chair of the Metalworking Fluid Management Education and Training Subcommittee. John Burke became the first Steering Committee chair, and John Howell became the first CMFS Examination Subcommittee chair.
We quickly changed the model for STLE course development. I recruited a team of experts to revise the Basic Metalworking Fluid Course. The subcommittee modified the existing course to ensure that the logical flow of the modules addressed the needs of fluid managers. We also vetted each module so that all course content represented the consensus of our expert team, rather than the perspective of individual presenters. We partnered with SME to present the course outside the annual meeting.
John Howell succeeded John Burke at the Steering Committee helm, and I followed John Howell. Earlier this year STLE President Dave Scheetz asked me to chair a new certification board. We held our inaugural meeting at the 2009 Annual Meeting & Exhibition in Lake Buena Vista, Fla. Our charter is to harmonize the CMFS, CLS and OMA certification committee processes and to ensure that we operate in conformity with ISO 17024, general requirements for bodies operating certification of persons.
TLT: Looking back at the beginning of your career, what has changed most about metalworking fluid microbiology?
Passman: When I first became involved with MWFs, the discipline was still quite new. Most of the early research focused on assessing the health risk posed by potentially pathogenic microbes that grew in MWFs.
By 1982, the consensus seemed to be that although potential pathogens were recovered routinely from MWF, no one was getting the diseases that these pathogens caused. The real issue was preventing the fluids from going bad. At that time, few plant operators made the connection between microbial contamination and fluid failure. As one plant manager told me: “Before you convinced us to monitor for microbes, we never had a microbial problem. We just dumped the system every eight to 12 weeks when the odor got so bad that the workers refused to stay in the plant.”
By the mid-1980s, EPA Clean Water Act regulations listed spent MWFs as hazardous waste. At the same time, several MWF compounders introduced the concept of fluid management. They recognized that well-maintained fluid could last for years (assuming normal fluid turnover during that period) without the need for a full system dump. They also recognized that if all else was managed properly (MWF concentration, water quality and particulate removal), uncontrolled microbial contamination was one of the most common causes of fluid failure.
At that time, I was doing a great deal of work on applications development for the catalase test. The method caught on quickly within the aluminum two-piece can industry where chemical managers had already been running tests for microbial contamination every shift. The 15-minute catalase test enabled operators to catch changes in microbial populations before they affected fluid performance.
From the mid-1980s through the late-1990s the primary focus was preventing microorganisms from causing fluid failure. This focus is obvious in my May 1988 Lubrication Engineering article,
Starting from Scratch: Microbial Problems in Metalworking Fluids.
However, after the sentinel hypersensitivity pneumonitis outbreak at an automotive plant in 1992, interest in MWF microbes as disease agents was renewed. As we entered the 21st Century, most companies involved with fluid management knew how to prevent fluid biodeterioration. However, the more pressing question became: “What role do MWF microbes play in allergenic diseases such as industrial asthma and hypersensitivity pneumonitis?” That’s where we are today.
There’s a growing body of data showing that airborne microbes and parts of microbes (bioaerosols) can cause respiratory disease. We don’t understand how bioaerosols interact with other aerosols present in MWF facilities. We don’t have a consensus on how best to protect exposed workers. We don’t even have consensus on what organisms or marker molecules we should be looking for. Consequently, we have returned to some of the same questions that were asked in the late-1940s when the first MWF microbiology research was done. This time around we are more interested in allergenic rather than communicable diseases.
TLT: What is being done to get the answers to these questions?
Passman: Right now is a particularly challenging time for the MWF industry. People with tremendous expertise, who have made great contributions to our knowledge over the years, are being retired or fired. The folks who remain behind at these companies are increasingly burdened with the workloads of their former colleagues. Travel funds are severely restricted. All of this translates into decreased opportunity for collaboration and applied research.
I hope the industry soon regains its momentum. Having said that, ASTM Subcommittee E.34.50, which I chair, is continuing to develop standard guides, practices and test methods to help industry stakeholders better understand risk factors and to better militate against those risks.
As I mentioned earlier, this year we have passed two new test methods: E2657
Method for Determination of Endotoxin in Water-Miscible Metalworking Fluids and E2694
Method for Measurement of Adenosine Triphosphate in Water-Miscible Metalworking Fluids (see Neil Canter’s Tech Beat article). Having also recently adopted two methods for enumerating
Mycobacterium immunogenum in MWFs, we are also working on a non-conventional method—polymerase chain reaction (PCR) for this microbe that has been implicated as one of the agents of hypersensitivity pneumonitis. The PCR methodology holds great promise for the future as a tool for fully characterizing MWF microbial populations.
Although culture methods (dip-slide or paddle tests) have served us quite well for more than 30 years, we don’t know whether there are medically important microbes that we aren’t detecting. New PCR techniques enable researchers to sort out all of the genetic material in a sample and determine the taxonomy of all of the microbes present.
In June 2009, ASTM approved a new standard: E2693
Practice for Prevention of Dermatitis in the Wet Metal Removal Fluid Environment. We are also beginning work on a recommended metalworking aerosol exposure limit. This last activity is particularly exciting because we’ll be recruiting representatives from fluid compounders, fluid managers, end-users, governmental agencies and unions to participate in the process.
There is a tremendous amount of work yet to be done. I outlined some of this in a paper that was published in
Tribology Transactions in January 2008 and reprinted in TLT in March 2008. It’s no coincidence that almost every member of E.34.50 is also a member of STLE. Since 2008 we have been holding an E.34.50 meeting in conjunction with the STLE Annual Meeting & Exhibition.
TLT: What do you see as the path ahead?
Passman: I believe the ASTM E.34.50 MWF Aerosol Recommended Exposure Limit Task Force, as it begins its work, will identify crucial knowledge gaps. By the time the Task Force has compiled the list of knowledge gaps, the industry will be well on its way to recovery and will support the work needed to close the knowledge gaps.
All industry stakeholders have a vest interest in developing a recommended exposure limit based on good science and adequately protective of worker health. The two key concepts here are good science—multidisciplinary studies that help us to better understand both the qualitative and quantitative relationships between exposure and disease risk—and
adequate. It’s unrealistic to try to create a zero-risk environment. Bioaerosols are present everywhere, including our houses, outdoors, commercial buildings and vehicles. We want to identify cost-effective measures that substantially reduce disease risk.
I am hopeful that over the course of the next decade we will make tremendous progress in this area. To help promote collaboration—particularly during times of tight travel restrictions—I recently formed an STLE MWF Microbiology Community on STLE’s Web site. I hope to use this as a forum for microbiologists and other STLE members who are interested in MWF microbiology. Through this community, we should be able to plan collaborative studies, share experiences and discuss the whole range of MWF microbiology.
Of course, my not-so-hidden agenda is that having devoted much of the past 26 years to MWF microbiology, I want to be sure that there will be others who will follow in my footsteps as I followed in the footsteps of Ed Bennett and Hal Rossmoore, colleagues and mentors who were responsible for drawing me into MWF microbiology.
Dr. Fred Passman recalls his start in microbiology research and offers a view on the future of MWF biocides.
THERMAL AND PH ENVIRONMENT
A number of bacterial species commonly found in metalworking fluids grow at pH 9.2-9.5. Like the facultative anaerobes, which create micro-environments for the anaerobes, these pH-tolerant bacteria create moderate pH environments for pH-sensitive microbes.
Microorganisms create “micro-environments” in which conditions may be very different than they are in the bulk fluid. These micro-environments are protected from changes in coolant temperature, chemistry and pH by the slime or glycocalyx matrix that the microbial population produces. In terms of pH, this means that even though a coolant may be maintained at pH 8.5-9.0, the pH within the microenvironment may be below 7.0. PH control often affects symptoms rather than the underlying problem of microbial growth.
CONCLUSIONS
Uncontrolled microbial growth can create problems ranging from gross odors and slimes to more subtle effects on coolant performance. All microorganisms require an energy source, nutrients and a suitable thermal and pH environment. The essence of coolant system management is optimizing operating conditions while keeping the environment of the metalworking fluid inhospitable for microorganisms. Worker health and safety considerations are of paramount importance in this equation.
Although it is beyond the scope of the present discussion to delve into health and safety questions associated with metalworking, a couple of points should be stressed. Metalworking fluids are complex mixtures of chemicals that are constantly changing due to the chemical, physical and microbiological factors which typify metalworking systems. Moreover, elevated concentrations of potentially pathogenic microorganisms are occasionally recovered from aerosols in metalworking plants. The risks posed by these conditions are poorly understood. The existing data suggest that good personal hygiene and health care minimize the risks due to coolant exposure in metalworking shops. People who have good personal-care habits do not seem to be at any greater health risk than any other portion of the general population.
POSTSCRIPT
Although 16 years have passed since I drafted the
Starting from Scratch article (
1), I remain comfortable with the fundamentals as I presented them in 1988. However, there are a few issues that can use some updating. At the time I wrote the article, there was little evidence of any relationship between microbial contamination and workplace disease. Although it still seems as though pathogenicity is a non-issue, there are recognized relationships between bioaersol exposure and respiratory disease. I have reviewed these in my 2002 article coauthored with professor Harold Rossmoore (
2).
In 1988 there was little talk about bioresistant additives. These are functional additives that are used to provide corrosion protection, lubricity or other performance property other than antimicrobial activity, that also render the fluid less readily biodegradable. During the past decade, there has been a proliferation of additives offered with bioresistance claims. Some of these products are valuable additions to the additive inventory. Others are simple unregistered microbicides for which no performance data exist. ASTM E2274 (
3), first published in 2003, provided details on how to evaluate metalworking fluid bioresistance. ASTM E2169 (
4) offers a through discussion of the issues that must be considered when selecting a metalworking fluid microbicide.
One fact amazes me as I reflect on how we monitor microbial contamination in metalworking fluids. Despite tremendous advances in microbiological methods, the industry continues to depend on techniques that were developed over a century ago. I’m not advocating that we toss out the baby with the bath water, but it might be time that metalworking fluid managers take advantages of some of the rapid microbiological methods that have found broad application in microbial ecology.
REFERENCES
1.
Passman, F.J. (1988), “Starting from Scratch: Microbial Problems in Metalworking Fluids,”
Lubrication Engineering,
44 (5), pp. 431-433.
2.
Passman, F.J. and Rossmoore, H.W. (2002), “Reassessing the Health Risks Associated with Employee Exposure to Metalworking Fluid Microbes,”
Lubrication Engineering,
58 (7), pp. 30-38.
3.
ASTM E2275,
Standard Practice for Evaluating Water-Miscible Metalworking Fluid Bioresistance and Antimicrobial Pesticide Performance, Annual Meeting Book of ASTM Standards Vol. 11.05, ASTM International, West Conshohocken, Pa.
4.
ASTM E2169,
Standard Practice for Selecting Antimicrobial Pesticides for Use in Water-Miscible Metalworking Fluids, Annual Meeting Book of ASTM Standards Vol. 11.03, ASTM International, West Conshohocken, Pa.
Fred Passman is founder and president of Biodeterioration Control Associates Inc. in Princeton, N.J. You can reach him at bcainc@comcast.net.